-
PDF
- Split View
-
Views
-
Cite
Cite
Nathan Wolf, Seth D. Newsome, Marilyn L. Fogel, Carlos Martinez Del Rio, The Relationship between Drinking Water and the Hydrogen and Oxygen Stable Isotope Values of Tissues in Japanese Quail (Cortunix japonica), The Auk, Volume 130, Issue 2, 1 April 2013, Pages 323–330, https://doi.org/10.1525/auk.2013.12075
- Share Icon Share
Abstract
Developing an understanding of how differences in the dietary ecology and physiology of different species can influence the incorporation of hydrogen and oxygen from resources into consumer tissues is an important factor to consider when designing, or interpreting data from, ecological studies using stable isotope analyses of these elements. Here, we present the results of an experiment designed to examine the relationship between the δ2H and δ18O of drinking water and the δ2H and δ18O values of body water, blood plasma, red blood cells, intestine, liver, muscle, and feathers of Japanese Quail (Cortunix japonica). Because Japanese Quail have high drinking-water requirements compared with many other bird species, we hypothesized that the relative contribution of drinking water to tissue δ2H and δ18O values would be higher than that of bird species with lower drinking-water requirements. Our results demonstrate that the contribution of drinking water to the δ2H and δ18O values of tissues is generally higher in Japanese Quail than in other birds with lower drinking-water rates. However, we failed to find significant relationships between drinking-water isotope values and tissue isotope values in many of the tissues that we examined. We suspect that this lack of significant relationships is the result of variation in tissue isotope values caused by differences in drinking-water consumption rates among individual birds. Given these results, we recommend that researchers use caution when interpreting data from ecological investigations using δ2H and δ18O analyses.
Resumen
Desarrollar un entendimiento sobre cómo las diferencias en la ecología de la dieta y la fisiologí a de diferentes especies pueden influir en la incorporación de hidrógeno y oxígeno en los tejidos de los consumidores a partir de sus recursos es un factor importante a considerar cuando se diseñan estudios ecológicos que usan análisis de isótopos estables de estos elementos o cuando se interpretan datos producto de tales estudios. Presentamos los resultados de un experimento diseñado para examinar la relación entre los isótopos δ2H y δ18O del agua consumida y los valores de δ2H y δ18O en agua corporal, plasma sanguíneo, glóbulos rojos, intestino, hígado, músculo y plumas de Cortunix japonica. Dado que C. japonica tiene altos requerimientos de toma de agua en comparación con muchas otras especies de aves, planteamos la hipótesis de que la contribución relativa del agua consumida a los valores de δ2H y δ18O en los tejidos serií mayor que la de especies de aves con requerimientos más bajos de consumo de agua. Nuestros resultados demuestran que la contribución del agua consumida a los valores de δ2H y δ18O en los tejidos generalmente es mayor en C. japonica que en otras especies de aves con requerimientos más bajos de consumo de agua. Sin embargo, no encontramos relaciones significativas entre los valores de isoóopos del agua consumida y de muchos de los tejidos que examinamos. Sospechamos que esta falta de relaciones significativas es el resultado de la variación en los valores de isótopos de los tejidos causada por diferencias en las tasas de consumo de agua entre los individuos. Dados estos resultados, recomendamos que los investigadores tengan cuidado al interpretar datos de investigaciones ecológicas que usan análisis de δ2H y δ18O.
USING HYDROGEN AND oxygen isotopes in animal ecology requires reliable estimates of the isotopic discrimination between animal tissues and local precipitation (Δ2Htissue-precipitation or Δ18O tisue-precipitation; Hobson 2005, 2008; Hobson et al. 2009). In a recent review, Hobson (2008) showed that great variability exists in both the goodness-of-fit and the slopes of the relationship between the δ2H of feathers and the δ2H of precipitation reported by observational studies. Hobson (2008) reported coefficient of determination (r2 ) values ranging from 0.36 to 0.91 (mean r2 = 0.55, n = 24) and slopes ranging from 0.28 to 1.41 (mean ± 95%CI = 0.80 ± 0.11, n = 27). This variation in both the degree of association and slope of δ2Hfeather versus δ2Hprecipitataion relationships has previously been attributed to variations in analytical techniques, including differences in laboratory protocols and inaccuracies in the modeling of the δ2H; values of precipitation (Lott and Smith 2006, Smith et al. 2009). However, the possibility that this variation may be attributable to differences in physiology or ecology among species has received less attention. One possible biological explanation for this variation that deserves further exploration is how differences in the dietary ecology of species may result in consequent variations in the relative contribution of the multiple sources from which the molecules (e.g., amino acids) that are used to synthesize proteinaceous animal tissues are derived (Wolf et al. 2011). These sources include food and preformed water (i.e., water in food and free drinking water) for hydrogen and food, preformed water, and atmospheric oxygen for oxygen (Hobson et al. 1999, 2009). Hobson et al. (1999) noted that variation in the isotopic composition of these sources could lead to variation in the relationship between the isotopic content of an animal's tissues and that of local precipitation. Another possibility is that individuals differ in the relative contribution of these sources to tissue hydrogen and oxygen. These differences can weaken the relationship between the isotopic content of an animal's tissues and that of local precipitation. This latter possibility has received relatively little attention.
Previous studies that have investigated the relative contributions of free drinking water and diet to the δ2H and δ18O values of avian tissues have reported variable results. Hobson et al. (1999) reported that 18–32% of the hydrogen in Japanese Quail (Cortunix japonica) tissues (blood, muscle, liver, lipids, feathers, and claws) was derived from preformed water. These results contrast with those of Wolf et al. (2011), who reported much lower contributions of preformed water (14%, 17%, and 18% of the hydrogen in red blood cells, plasma, and feathers, respectively; and 15% and 27% of the oxygen in red blood cells and feathers, respectively) to the tissues of House Sparrows (Passer domesticus). Wolf et al. (2011) speculated that the lower preformed water contributions might be the result of the low drinking-water requirements and small-drinking water uptake of granivorous birds, such as House Sparrows (MacMillen 1990), compared to those with higher water intake, such as Japanese Quail (Bartholomew and MacMillen 1961). Unfortunately, Hobson et al. (1999) and Wolf et al. (2011) used slightly different methods to determine the fractional contributions of drinking water to the tissue isotopic values of the birds in their studies. Given the difference between the two studies, we cannot reliably determine whether the difference in the fractional contribution of drinking water to consumer tissues reported by Hobson et al. (1999) and Wolf et al. (2011) is, indeed, the result of a physiological or ecological mechanism and not of differences in experimental protocol.
Here, we present the results of a study designed to reexamine the relationship between drinking-water δ2H value and the δ2H values of Japanese Quail tissues (body water, plasma, red blood cells, intestinal muscle, liver, skeletal muscle, and feathers) using the same methods used by Wolf et al. (2011) to examine this relationship in House Sparrows. Our experiment also allowed us to examine the relationship between the isotopic value of preformed drinking water and the δ18O signatures of these same seven Japanese Quail tissues, which was not examined by Hobson et al. (1999). This examination will aid in our understanding of the causes of variation seen in the relationship between the isotopic signature of tissues and that of local precipitation in birds (Hobson 2008). Such an understanding will, in turn, allow us to design and interpret data from ecological studies that use δ2H and δ18O analysis more precisely and confidently. A secondary goal of our work was to determine the variation in the δ2H and δ18O values among the seven Japanese Quail tissues listed above. On the basis of previous observations that discrimination values for hydrogen and oxygen can vary greatly between tissues (Wolf et al. 2011), we expected the tissue-to-drinking-water discrimination factors to differ among the seven Japanese Quail tissues examined. These tissue-specific discriminations have significant implications for comparisons between ecological studies that analyze different tissues, and serve to emphasize the need for accurate tissue-specific discrimination values when attempting to use hydrogen or oxygen isotopes to study animal movements. By exploring the differences in these aspects of the relationship between consumer tissues and drinking water for two birds with different drinking-water needs, we can develop a more complete understanding of the mechanisms that shape the relationship between the δ2H and δ18O values of resources and those of consumer tissues.
Methods
Fifteen adult Japanese Quail (6 males and 9 females, body mass [Mb] = 176 g) were housed at 26.0°C in individual cages (40 × 30 × 25 cm) at the University of Wyoming Department of Zoology and Physiology Animal Care Facility (Laramie, Wyoming). The birds were kept on an initial diet of Common Wheat (Triticum aestivum, δ2H = -85‰, δ18O = 25‰) and provided with tap water (δ2H = -132‰, δ18O = -17‰) ad libitum until their red blood cells and blood plasma achieved an asymptotic value that reflected that of the food and water (90 days). The birds also received a vitamin and mineral supplement (Nekton S, Nekton GmbH, Pforzheim, Germany). Following this 90-day equilibration period, the birds were randomly assigned to one of three treatment groups of five birds each. The three treatment groups continued to receive the same Wheat diet (and vitamin supplement), but each group was provided with a drinkingwater treatment with different δ2H and δ18O values [δ2H (δ18O) = -111(-15)‰ -87(-13)‰, -58(-11)‰]. The δ2H and δ18O of the drinking water in each treatment was created by adding 99 atom percent deuterated water (D2O) and 98 atom percent 18O water (H2O18) to distilled local tap water. Both diet and drinking-water sources were purchased and mixed in large volumes to ensure isotopic consistency. The variation in the isotopic values of the three water treatments was designed to approximate the natural variation in environmental waters along the Global Meteoric Water Line (Craig 1961).
Following the drinking-water switch, drinking-water consumption of individual Japanese Quail was measured (mL) every 2 days, and blood samples (∼0.5 mL) were taken from each bird on days 0, 2, 4, 8, 16, 32, 64, and 90. Blood samples were taken from the brachial vein using a 0.5-mL syringe equipped with a 30-gauge needle, and centrifuged (2 min in a microhematocrit centrifuge) to separate red blood cells and plasma. All blood and tissue samples were collected at the same time of day (∞1300 hours Mountain Standard Time). Body water was cryodistilled from plasma using the method described by Ehleringer (1989). Red blood cells were sampled over time to determine whether the isotopic value of the birds' blood had reached a constant asymptotic value reflecting that of the drinking water. After 90 days, several primary feathers were plucked from each bird and allowed to regrow. The newly grown feathers were sampled to examine the relationship of drinking-water δ2H and δ18O and tissue δ2H and δ18O in feathers, a common tissue used in isotope-based studies of avian movement. Following feather sampling (day 104), birds were euthanized using CO2 asphixiation, and intestine, liver, and muscle samples were collected. We chose these tissues on the basis of their prevalence in the isotope literature (Carleton et al. 2008, Caut et al. 2009). Tissue samples were stored frozen at -80°C until processing.
All tissue samples except feathers were dried to a constant mass in an oven at 5.0°C and ground into a fine powder using a mortar and pestle. Feathers were rinsed with distilled water and air dried. Paritte and Kelly (2009) have explored the implications of different feather-cleaning methods δ2H values. They concluded that the best method for cleaning feathers is a 2:1 chloroform methanol rinse, followed by a detergent rinse. Although we recognize that this may be the most effective method for feather cleaning prior to hydrogen stable isotope analysis, we chose to use a simple distilled-water rinse because this is the same method used by Wolf et al. (2011) to prepare House Sparrow feathers. Using surgical scissors, we removed the feather barbs from the rachis and minced and homogenized the barbs; thus, samples for isotope analysis did not include the rachis. All powdered or minced tissue samples were then soaked in three 24-h treatments of petroleum ether to remove lipids. Following lipid removal, two randomly selected subsamples of each tissue sample were loaded into silver capsules (mean aliquot mass = 0.14 ± 0.03 mg; Costech Analytical Technologies, Valencia, California) for hydrogen and oxygen stable isotope analyses. In order to control for exchangeable hydrogen, encapsulated samples were equilibrated for at least 2 weeks prior to analysis in a 50°C oven under a constant stream of N2 alongside keratin reference materials (Domestic Chicken [Gallus gallus] and Domestic Turkey [Meleagris gallopavo] feathers) with known non-exchangeable δ2H values (chicken feather: δ2H = -92 ± 2.0‰ and turkey feather: δ2H = -49 ± 2.0‰). These reference materials were calibrated to the non-exchangeable hydrogen of a whale baleen laboratory standard ( δ2H = -108‰) at Environment Canada. Measured δ2H values of the chicken and turkey feather values were compared with their known non-exchangeable δ2H values. The observed relationship between the measured and known non-exchangeable δ2H values of these reference materials was used to adjust for the effect of exchangeable hydrogen (Britzke et al. 2009) in unknown samples. Despite the varied natures and large potential range of δ2H values of the tissues we analyzed, we used these reference materials for all analyses because of a lack of availability of specific reference materials for other tissues. The δ2H values were determined using a Finnigan TC/EA (Thermo Fisher Scientific, Waltham, Massachusetts) coupled to a ThermoFinnigan Delta Plus XL mass spectrometer (Thermo Fisher Scientific) at the Carnegie Institution of Washington (Washington, D.C). Samples were introduced into the TC/EA using a Costech Zero Blank autosampler. While in the autosampler, possible hydrogen exchange between ambient water vapor and samples was controlled-for by flooding the sealed autosampler carousel with He. In addition, all samples were equilibrated and analyzed using the same methods during the same period. Analysis of the two keratin and two oil (pump oil and mineral oil) reference materials showed that the precision of δ2 Hanalysis was ≤4‰. Prior to δ18O analysis, tissue materials were equilibrated alongside protein reference materials (beef blood, δ18O = 6 ± 0.3‰; beef liver, δ18O = 8 ± 0.5‰; and feather keratin, δ18O = 8 ± 0.3‰) for at least 2 weeks. The δ18O values were determined using a Thermo Finnigan TC/EA coupled to a Thermo-Finnigan Delta Plus XP continuousflow mass spectrometer (Thermo Fisher Scientific) at the University of Wyoming Stable Isotope Facility. Analysis of reference materials (beef blood, beef liver, feather keratin, and benzoic acid) showed that the precision of δ18O analysis was <0.5‰. The δ2H and δ18O values of liquid water and body water cryodistilled from soft tissues were determined using the Los Gatos Research (LGR) Laser Water Analyzer (Los Gatos Research, Mountain View, California) at the University of Wyoming Stable Isotope Facility.
To assess whether the isotope values of bird tissues had achieved constant asymptotic values, the delta values of red blood cells from repeated sampling events were compared using paired t-tests. Following Ehleringer et al. (2008), Bowen et al. (2009), and Wolf et al. (2011), we used standard least-squares linear regression to establish the relationship between the isotopic composition of individual tissues and that of drinking water. When the isotopic signatures of consumer tissues had a linear relationship to drinking-water isotopic signatures, we used the slope of the relationship as a first-order estimate of the fractional contribution of drinking water to the hydrogen and oxygen content of tissues (Ehleringer et al. 2008). Differences in the isotopic values among tissues were analyzed using multivariate analysis of variance (MANOVA) with treatment, tissue, and treatment * tissue as independent variables and isotopic value as a response variable. In cases where tissue values were missing, the entire bird was removed from the MANOVA. Post hoc comparisons among means for all MANOVAs were done using Tukey's HSD tests. Differences in tissue stable isotope values between sexes and drinkingwater consumption volume levels were analyzed using repeatedmeasures analysis of variance (RM ANOVA). We used RM ANOVA because tissues sampled from the same bird are not statistically independent. By using RM ANOVA, we were able to compare the δ2H or δ18O values among the tissues without violating any test assumptions. For each RM ANOVA, we completed a Mauchly's test for sphericity to ensure that all possible pairs of groups met the RM ANOVA's assumption that variances of groups were equal. If this test was statistically significant, we used the Greenhouse-Geisser corrected values for RM ANOVA. The Greenhouse-Geisser correction is a conservative technique that prevents the Type I error associated with sphericity (Zar 1996). In cases where tissue values were missing, the entire bird was removed from the RM ANOVA analysis. All statistical analyses were performed in the program JMP, versions 9.0 and 10.0 (SAS Institute, Cary, North Carolina).
Results
In the 90 days following the drinking-water switch, the birds did not experience a significant change inbodymass (initial mean Mb = 176 g, final mean Mb = 174g, t = 1.71, df =14, P = 0.06). Mean daily drinking-water volumes for individual birds were relatively high and displayed high variability (total daily average = 36.78 ± 15.38 mL [approximately 21% ± 8% of Japanese Quail Mb). One bird from treatment group 3 died before the end of the experiment. We did not include any tissues from this bird in our analyses. We were also unable to collect feathers from one bird in treatment group 3 because of a lack of feather regrowth. In addition, we were unable to collect day-64 red blood cells, plasma, and body water samples from one bird in treatment group 2, and we lost day-64 plasma samples for δ2H for one bird from treatment group 3 and for for δ18O for one bird from treatment group 1 because of autosampler error. Within each treatment, the delta values of red blood cells reached constant values by day 32 for both hydrogen (t = 1.4, df = 12, P = 0.18) and oxygen (t = 0.8, df = 12, P = 0.38).
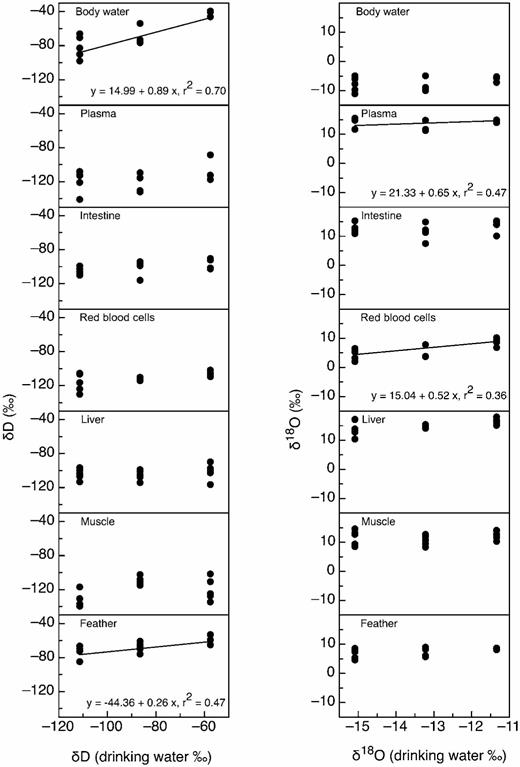
Relationships between the δ2H (left) and δ18O (right) of drinking water and that of seven tissue types in Japanese Quail: body water, blood plasma, intestine, red blood cells, liver, muscle, and feathers. Regression lines are provided for significant relationships. The δ2H values of body water and feathers were significantly correlated with those of drinking water. The δ18O values of red blood cells and plasma were significantly correlated with those of drinking water.
The δ2H values of body water and feathers were linearly related to the δD values of drinking water (Fig. 1). However, the δ2H values of plasma, red blood cells, intestine, liver, and skeletal muscle, did not show a statistically significant linear relationship with those of drinking water (Fig. 1). The estimated percent contributions (± SE) of drinking water to the hydrogen isotopic signatures of tissues were 89 ± 17% for body water and 26 ± 8% for feathers. For oxygen, red blood cells and plasma were the only tissues for which the δ18O values were significantly linearly related to the δ18O values of drinking water (Fig. 1). No other tissue had δ18O values that displayed significant relationships with those of drinking water (Fig. 1). The estimated percent contribution (± SE) of drinkingwater to the oxygen isotopic signatures of tissues was 52 ± 20% for red blood cells and 65 ±21% for plasma.
Mean (± SD) isotopic values of Japanese Quail tissues in three drinking water treatments. Within treatments, these means varied significantly. For each treatment, tissues are arranged from the lowest isotopic value to the highest. Tissues with different letters are significantly different (Tukey's HSD, P < 0.05).
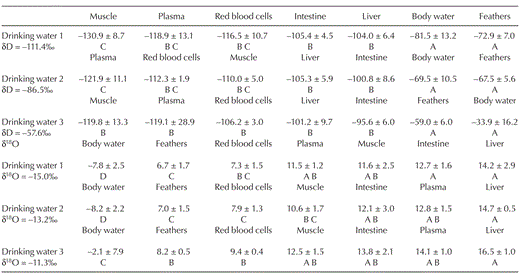
Mean (± SD) isotopic values of Japanese Quail tissues in three drinking water treatments. Within treatments, these means varied significantly. For each treatment, tissues are arranged from the lowest isotopic value to the highest. Tissues with different letters are significantly different (Tukey's HSD, P < 0.05).
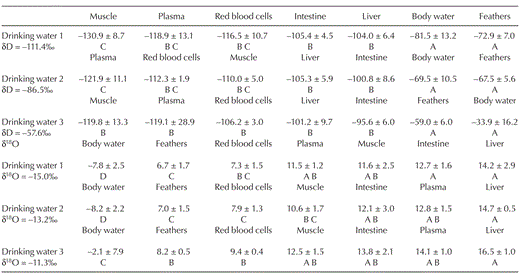
We found significant differences in the isotopic values among tissues for hydrogen (MANOVA, F = 4.088, df = 7 and 88, P < 0.0001; Table 1) but no significant interaction between tissue type and drinking-water treatment group for hydrogen (MANOVA, F = 0.28, df = 14 and 88, P = 0.051). The δ2H values of feathers and body water were more enriched in deuterium than other tissues, whereas muscle and blood plasma tended to be more depleted than other tissues (Table 1 and Fig. 2). There was no significant effect of sex (uncorrected RM ANOVA, F = 0.30, df = 6 and 42, P = 0.93) or daily drinking-water volume (uncorrected RM ANOVA, F = 0.24, df = 6 and 42, P = 0.24) on tissue δ2H values. We also found significant differences in the isotopic values among tissues for oxygen (MANOVA, F = 14.96, df = 7 and 82, P < 0.0001; Table 1 and Fig. 2), but no significant interaction between tissue type and drinking-water treatment group for oxygen (MANOVA, F = 0.12, df = 14 and 82, P = 0.74). Body water was significantly more depleted in 18O than other tissues, and liver was significantly more enriched in 18O than other tissues (Table 1 and Fig. 2). There was no effect of sex (Greenhouse-Geisser corrected RM ANOVA, F = 0.57, df = 1.50 and 9.03, P = 0.53) or daily drinking-water volume (Greenhouse-Geisser corrected RM ANOVA, F = 0.21, df = 1.50 and 9.03, P = 0.74) on tissue δ18O values.
The δ2H and δ18O values were significantly correlated in body water (r = 0.837, P < 0.05) and feathers (r = 0.700, P < 0.05; Fig. 3). There were no significant correlations between δ2H and δ18O values in any other tissue (Fig. 3).
Discussion
Estimating the contribution of drinking water to tissue δ2H and δ18O signatures usingslopes.— Given Hobson et al.'s (1999) results, we expected the slopes of the relationships between drinking-water δ2H values and Japanese Quail tissue δ2H values to be greater than those found in House Sparrows (Wolf et al. 2011). For hydrogen, this expectation proved correct for body water and feathers. Both of these tissues displayed significant linear relationships between tissue and drinking-water δ2H that indicated drinkingwater contributions of 26% and 89% for feathers and body water, respectively. These estimates are similar to those found by Hobson et al. (1999). By contrast, for House Sparrows, drinking-water contributions to tissue δ2H values ranged between 14% and 18% (Wolf et al. 2011). For the majority of the Japanese Quail tissues in our experiment, however, no significant relationships between the hydrogen isotopic signatures of tissues and those of drinking water were observed.
For oxygen, significant linear relationships between tissue and drinking-water δ18O were observed for red blood cells and plasma. Previous studies (O'Brien and Wooller 2007, Ehleringer et al. 2008, Wang et al. 2009, Nielson and Bowen 2010, Wolf et al. 2011) using a variety of tissues have reported larger contributions of drinking water to the oxygen in tissues than those observed for the hydrogen in tissues. These contributions for oxygen range from 15% in House Sparrow feathers (Wolf et al. 2011) to 70% in the chitin produced by larval chironomids and brine shrimp (Wang et al. 2009, Nielson and Bowen 2010). Our estimates of a 50% contribution of drinking water for red blood cells and a 65% contribution for plasma are both well within this general range, and higher than the estimated contributions of drinking water to the δ18O values of House Sparrow tissues reported by Wolf et al. (2011).
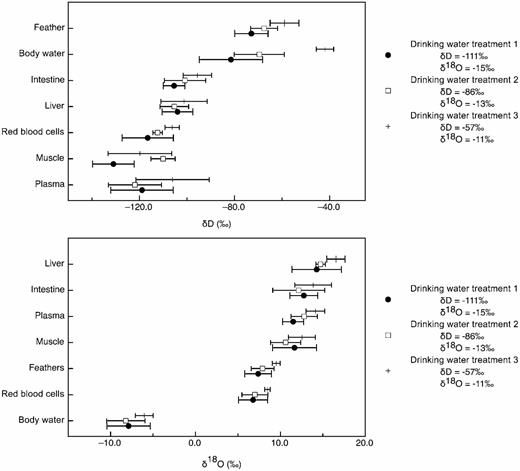
Mean (± SE) of δ2H and δ18O values for each tissue type from Japanese Quail in three drinking-water treatments. We found significant differences in the isotopic values between tissues for both hydrogen and oxygen. There was no significant interaction between tissue type and drinkingwater treatment group for either isotope. There was no significant effect of treatment or sex.
Our results demonstrate that the mechanism behind the difference in the fractional contributions of drinking water to tissue isotopic signature between Japanese Quail (Hobson et al. 1999) and House Sparrows (Wolf et al. 2011) remains unknown. Ehleringer et al. (2008) and Wolf et al. (2011) have demonstrated that the slopes of the relationships between drinking-water δ2H or δ18O and tissue δ2H or δ18O are likely dependent on the proportion of body-water hydrogen or oxygen derived from drinking water. Thus, the relatively low contribution of drinking water to tissue δ2H and δ18O values in House Sparrows as compared with Japanese Quail likely results from the differences in water requirements of the two species (Bartholomew and MacMillen 1961, MacMillen 1990). The influence of drinking-water intake on the fractional contribution of drinking water to tissue isotopic values would also explain the larger contribution of drinking water to tissue δ18O than to tissue δ2H values. Because the oxygen atoms in the carboxylic acid group of amino acids are exchanged with oxygen atoms from the body-water pool during digestion and proteolysis (Bernlohr 1972), a large proportion of the oxygen atoms in the amino acids comprising the animal's tissues will be derived from drinking water for animals in which the proportion of drinking water in the body-water pool is greater than that of metabolically produced water.
The small number of tissues for which a significant linear relationship existed between tissue isotopic signature and drinking-water isotopic signature (Fig. 1) is surprising given the success of this technique in previous studies (Ehleringer et al. 2008, Bowen et al. 2009, Wolf et al. 2011). This unexpected result may be a consequence of the multiple sources from which the hydrogen and oxygen in animal tissues can be derived: food and preformed water (i.e., water in food and free drinking water) for hydrogen; and food, preformed water, and atmospheric oxygen for oxygen (Hobson et al. 1999, 2009). The interaction and relative contributions of these sources influence the final isotopic value of consumer tissues. Consequently, variations in the input of one of these potential sources can significantly influence the final isotopic value of the consumer tissue.
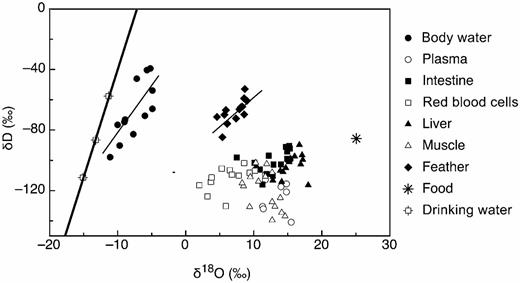
The δ2H and δ18O values of body water and feathers were positively and linearly related (y = -16.43 + 6.80x, r2 = 0.60 and y = -94.79 + 3.74x, r2 = 0.49 for body water and feather, respectively; P < 0.01 ). The δ2H and δ18O values of no other tissues were statistically related. For reference, we included the meteoric water line and the isotopic composition of food.
Variation in the isotopic signatures among tissues.—The large amount of variation we observed in the isotopic composition of tissues was similar to that found in House Sparrows (Wolf et al. 2011). The processes that contribute to variation in isotopic discrimination among tissues are well studied for carbon and nitrogen isotopes (Martínez del Rio et al. 2009). These include nutrient routing, differences in protein turnover, and differences in the amino acid compositions of tissues (Martínez del Rio et al. 2009). We suspect that the differences in the δ18O and δ2H values of tissues are also the result of these processes. Amino acid composition can vary greatly among tissue types within an individual. For example, bird feathers have ∼1,400 µmoles g-1 dry mass of glycine and 540 µmoles g-1 dry mass of glutamic acid (Murphy et al. 1990). Bird muscle, on the other hand, has only ∼798 µmoles g-1 dry mass of glycine but has ∼1,115 µmoles g-1 dry mass of glutamic acid (Murphy 1994). Because the δD value of different amino acids can differ by >300‰ in a single tissue (M. L. Fogel et al. unpubl. data), differences in the amino acid composition of tissues are likely the primary cause for observed variation in the discrimination values among tissues (Fig. 2).
Implications for the use of δ2H and δ18O analysis in the laboratory and field.— Our results have several implications for the use of δ2H and δ18O analysis in ecological studies and for the interpretation of δ2H and δ18O data. First, it appears that the differences in the fractional contribution of drinking water to tissue δ2H and δ18O signatures observed by Hobson et al. (1999) and Wolf et al. (2011) are the result of interspecific differences in physiology and/or ecology and are not due to differences in experimental or laboratory protocol (Lott and Smith 2006, Smith et al. 2009). In Japanese Quail, the coefficient of determination of the relationship between the δ2H of feathers and the δ2 H of precipitation was 0.47, and the slope of this relationship was 0.25. For comparison, using the same experimental protocol, Wolf et al. (2011) found a coefficient of determination of 0.73 and a slope of 0.18 for this same relationship for House Sparrow feathers. These differences in the strength and slope of the relationships between the δ2H values of drinking water and the δ2H values of Japanese Quail and House Sparrow feathers suggest that interspecific differences in the mechanisms that influence the transfer of hydrogen from resources to consumer tissues may play an influential role in creating the variation reported by Hobson (2008). Although differences in water intake seem a likely candidate for this mechanism, more specific research is needed to confirm this suspicion.
Our research also showed that despite an isotopically uniform diet, significant differences in δ2H and δ18O values can occur among tissues from the same individual. Because of these differences, we urge caution when considering comparisons between different tissue types. Analyses using δ2H and δ18O are a powerful technique for studying animal ecology. Unlike carbon and nitrogen, however, the pathway(s) from resources to consumer tissues is not necessarily straightforward for hydrogen and oxygen. Consequently, researchers who wish to use δ2H or δ18O analysis to study animal ecology should recognize this complexity and interpret the results of such investigations conservatively.
Acknowledgments
We thank R. Carroll for his assistance with bird care and R. Bowden for her assistance with δD analysis. N. Wolf was supported by the University of Wyoming Dr. George E. Menken's Memorial Scholarship. C. Martinez del Rio and S. D. Newsome were supported by National Science Foundation DIOS 0848028. M. L. Fogel was supported by the Carnegie Institution of Washington and the W. M. Keck Foundation.
Literature Cited
Author notes
Associate Editor: M. Klaassen