-
PDF
- Split View
-
Views
-
Cite
Cite
Heather R. L. Lerner, Matthew C. Klaver, David P. Mindell, Molecular Phylogenetics of the Buteonine Birds of Prey (Accipitridae), The Auk, Volume 125, Issue 2, 1 April 2008, Pages 304–315, https://doi.org/10.1525/auk.2008.06161
- Share Icon Share
Abstract
Phylogenetic relationships among birds of prey in the subfamily Buteoninae are not fully established but are of particular interest because the Buteoninae constitute one of the largest accipitrid subgroups and include multiple species of conservation concern. Genera previously included within the Buteoninae are Buteo, Leucopternis, Buteogallus, Harpyhaliaetus, Busarellus, Parabuteo, Geranoaetus, Geranospiza, Ictinia, Rostrhamus, Kaupifalco, and Butastur. We analyzed representatives from all buteonine genera and most non-Buteo (i.e., "sub-buteo") species with >3,000 bases of nuclear and mitochondrial DNA and found non-monophyly for the nominal genera Buteo, Buteogallus, and Leucopternis. The Old World Lizard Buzzard (Kaupifalco monogrammicus) is not closely related to buteonine taxa but is sister to goshawks in the genera Melierax, Micronisus, and Urotriorchis. Another Old World genus, Butastur, is sister to the clade including all other buteonine genera mentioned above. Investigation of several "superspecies" complexes within the genus Leucopternis revealed non-monophyly for the four subspecies of White Hawk (L. albicollis). On the basis of mitochondrial data, L. a. albicollis forms a clade with L. polionotus, whereas L. a. costaricensis, L. a. ghiesbreghti, and L. a. williaminae form a clade with L. occidentalis. Among taxa included as outgroups, we found two species in the genus Circus to be clearly nested within a clade of Accipiter spp.
Filogenética Molecular de las Aves de Presa Buteoninas (Accipitridae)
Resumen.—Las relaciones filogenéticas entre las aves de presa de la subfamilia Buteoninae no están completamente establecidas, pero son de particular interés porque éste es uno de los grupos más grandes de accipítridos, e incluye varias especies de interés en conservación. Los géneros incluidos previamente en Buteoninae son Buteo, Leucopternis, Buteogallus, Harpyhaliaetus, Busarellus, Parabuteo, Geranoaetus, Geranospiza, Ictinia, Rostrhamus, Kaupifalco y Butastur. Analizamos representantes de todos los géneros de buteoninos y la mayoría de especies que no pertenecen al género Buteo (i.e., "sub-buteos") con más de 3,000 bases de ADN nuclear y mitocondrial, y documentamos la no monofilia del género nominal Buteo, de Buteogallus y de Leucopternis. La especie del Viejo Mundo Kaupifalco monogrammicus no está cercanamente emparentada con taxones buteoninos, y forma el grupo hermano del clado formado por los géneros Melierax, Micronisus y Urotriorchis. Otro género del Viejo Mundo, Butastur, es hermano del clado que incluye todos los demás géneros de buteoninos antes mencionados. La investigación de varios complejos de "superespecies" dentro del género Leucopternis reveló que las cuatro subespecies de L. albicollis no forman un grupo monofilético. Con base en los datos mitocondriales, L. a. albicollis forma un clado con L. polionotus, mientras que L. a. costaricensis, L. a. ghiesbreghti y L. a. williaminae forman un clado con L. occidentalis. Entre los taxones incluidos como grupos externos, encontramos que dos especies del género Circus están claramente anidadas dentro de un clado formado por especies de Accipiter.
One of the largest groups in the family Accipitridae, the subfamily Buteoninae includes 24 "sub-buteo" species (Amadon 1982), two genera of kites (Lerner and Mindell 2005), and 25–28 species in the genus Buteo (Ferguson-Lees and Christie 2001, Dickinson 2003). The Buteoninae are of particular interest, because 11 species are of conservation concern (IUCN 2007), with one critically endangered species (Buteo ridgwayi) and two endangered species (Leucopternis occidentalis and Harpyhaliaetus coronatus). This subfamily also has included the sea and booted eagles (Grossman and Hamlet 1964) or the sea, booted, and harpy eagles (Friedmann 1950). Our recent molecular analysis, however, showed that the sea, booted, and harpy eagles form monophyletic groups separate from a clade of 10 sub-buteos, two kites, and three species in the genus Buteo (Lerner and Mindell 2005). Therefore, we do not consider any of the eagle groups members of Buteoninae. For the purposes of the present study, we consider Buteoninae to comprise Buteo and the nine sub-buteo and two kite genera previously proposed as, or found to be, close relatives of Buteo: New World hawks Leucopternis, Buteogallus (including Heterospizias), Harpyhaliaetus, Busarellus, Parabuteo, Geranoaetus, Asturina (now Buteo), and Geranospiza; Old World hawks Kaupifalco and Butastur (Amadon 1982); and the kites Ictinia and Rostrhamus (Lerner and Mindell 2005).
Although polyphyly of the sub-buteo group with respect to Buteo has long been suspected, only recently has it been shown that the genera Buteo, Leucopternis, and Buteogallus are not monophyletic with respect to each other (Riesing et al. 2003, Lerner and Mindell 2005, do Amaral et al. 2006). The full extent of polyphyletic relationships in Buteoninae is not known, because not all nominal species and subspecies have been included in a single analysis. Furthermore, previous analyses have not tested phylogenetic relationships in the Buteoninae in the context of the other major accipitrid clades. In particular, the placement of the Lizard Buzzard (Kaupifalco monogrammicus), Black-collared Hawk (Busarellus nigricollis), and genus Butastur remains to be assessed with molecular data in the broader context of the Accipitridae. The three species of Butastur have not previously been included in peer-reviewed analyses of molecular data sets. Neither Kaupifalco nor Busarellus formed close sister relationships with three other sub-buteo genera, 25 Buteo species, a booted eagle, and an accipiter in a study by Riesing et al. (2003) using mitochondrial NADH dehydrogenase subunit 6 (ND6) and pseudo-control region sequences. With a phylogeny generated from 191 osteological characters for 44 accipitrid taxa, Holdaway (1994) did not find a close relationship between Kaupifalco and any other accipitrid species. In the same study, Busarellus was sister to booted eagles Hieraaetus and Polemaetus, though nodal support values were not presented for the phylogeny.
Species status has been questioned for taxa in the sub-buteonine genera Buteogallus (B. anthracinus, B. subtilis, and B. aequinoctialis) and Leucopternis (L. schistaceus and L. plumbeus; L. kuhli and L. melanops; and L. albicollis, L. occidentalis, and L. polionotus) where widespread taxa occupying similar niches have been divided into multiple subspecies or separate species without conclusive evidence one way or the other (Amadon 1982). A recent mitochondrial phylogeny found sister relationships for L. kuhli, L. melanops, and L. albicollis, and for L. occidentalis and L. polionotus, but not for L. schistaceus and L. plumbeus (do Amaral et al. 2006). Still, the other questioned groups in Leucopternis and Buteogallus have not been tested with molecular data, and further testing of most of these groups is needed to evaluate current taxonomy.
A comprehensive analysis of the phylogenetic relationships among proposed buteonine genera and species is needed to address remaining questions about the group's evolutionary history. With complete taxonomic representation of genera and nearly all nominal species and subspecies of sub-buteos, we address four questions. (1) Are Kaupifalco, Busarellus, and Butastur closely related to other proposed buteonines? (2) What are the sister relationships among genera in Buteoninae? (3) To what extent are the genera polyphyletic? (4) Is there evidence of genetic divergence and reciprocal monophyly to support current taxonomy for species and subspecies of Buteogallus and Leucopternis?
Methods
We sampled at least one individual of each nominal genus and species, and nearly all subspecies, of sub-buteos. Our final sampling included 107 individuals representing 45 out of 55 buteonine species, 26 out of 176 non-buteonine accipitrid species, and 2 non-accipitrid outgroup species (Ferguson-Lees and Christie 2001, Dickinson 2003; Table 1). To test monophyly of Buteoninae, we included representatives of each recognized subfamily or primary clade of Accipitridae. We also included multiple representatives of Circus, Melierax, and Accipiter and one sample each for two monotypic genera (Micronisus and Urotriorchis) on the basis of finding a close relationship between these taxa and Kaupifalco using published ND2 and cytochrome-b (cyt-b) sequences (Lerner and Mindell 2005). To incorporate more Buteo spp. in our analyses and to compare our results with two recent molecular studies, we also sequenced ND6 from 42 of our buteonine samples and analyzed them in a data set with previously published ND6 sequences from an additional eight Buteo spp., eight Buteo subspecies, and three non-Buteo buteonine subspecies (Riesing et al. 2003, do Amaral et al. 2006; Table 1). Samples were identified to the subspecies level on the basis of specimen labels or collection locality. Common names follow the 7th edition of the Check-list of North American Birds and its supplements (American Ornithologists' Union [AOU] 1988) or the Handbook of Birds of the World (Thiollay 1994).
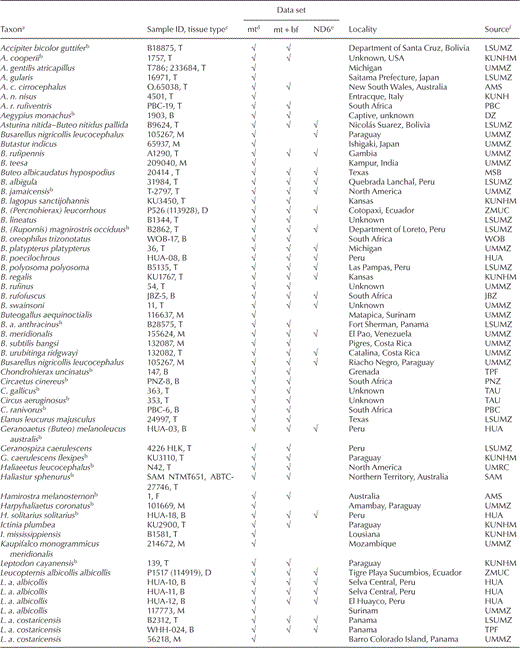
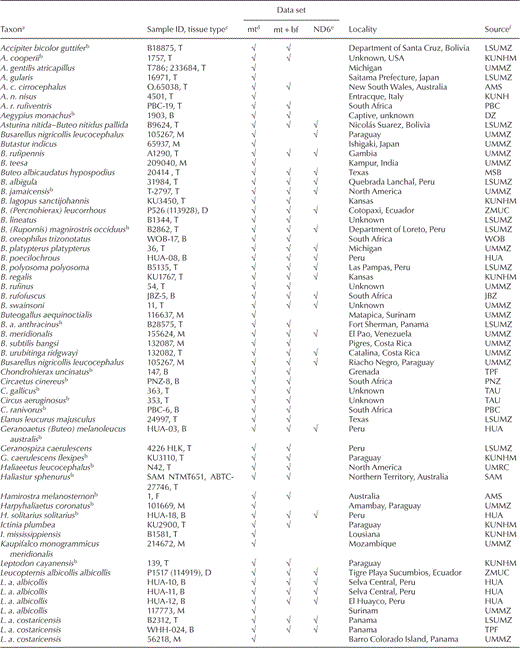
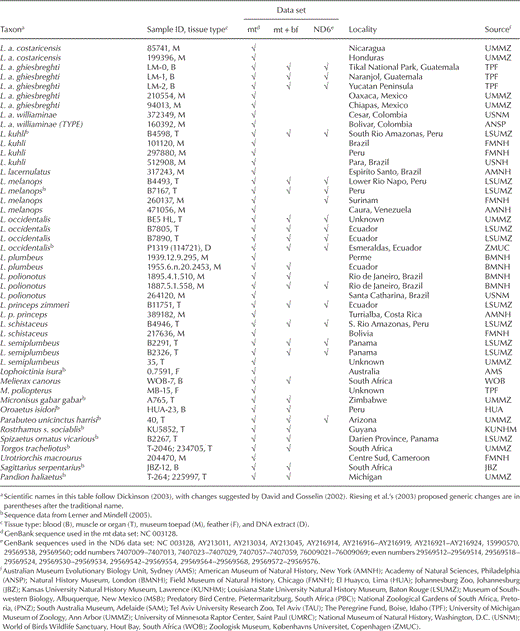
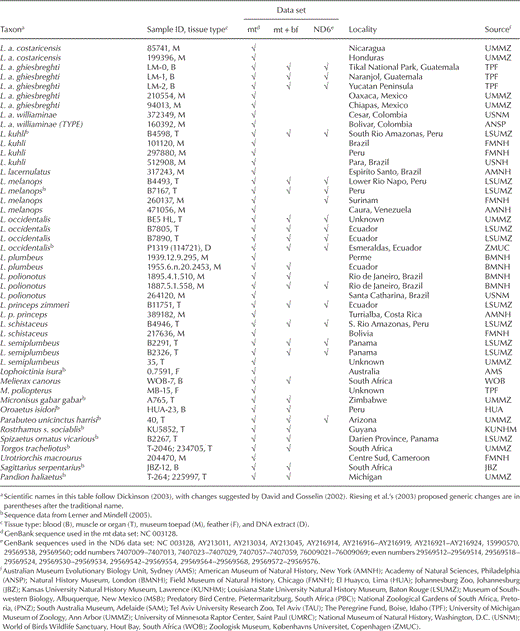
Total genomic DNA was extracted from blood or other tissue of contemporary specimens or from toe-pad tissue of museum specimens using a DNeasy tissue extraction kit (Qiagen, Valencia, California). Lab work involving DNA extraction and polymerase chain reaction (PCR) set up from museum samples was conducted in a facility reserved for ancient DNA at the University of Michigan Museum of Zoology using protocols developed for ancient DNA, including multiple extraction and PCR controls (Cooper and Poinar 2000). The PCR amplifications were conducted using primers we designed for the Buteoninae, as well as published primer sequences for avian mitochondrial cyt-b, ND2, ND6, the nonrepetitive part of the pseudo-control region, and nuclear BF-I7 (primer sequences are reported in Table 2). These genomic regions were chosen for their ability to resolve both recent and deep divergences and their comparability with published sequences (Sorenson et al. 1999, Prychitko and Moore 2000, Riesing et al. 2003, Lerner and Mindell 2005, do Amaral et al. 2006). The PCR products were gel purified using a QIAquick gel extraction kit (Qiagen), directly sequenced from both strands with ABI big dye terminator chemistry, and resolved on an ABI 3730 automated sequencer. Sequences were viewed as chromatographs in SEQUENCHER, version 4.5 (Gene Codes, Ann Arbor, Michigan), and aligned by eye in BIOEDIT sequence alignment editor (Hall 1999).
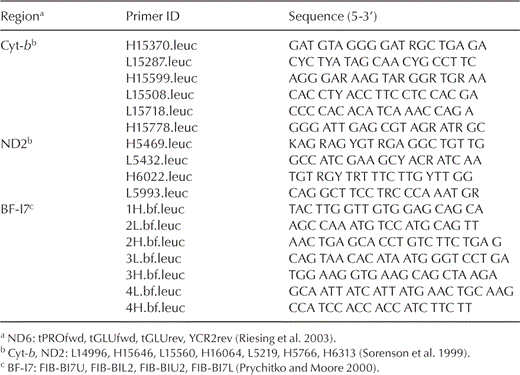
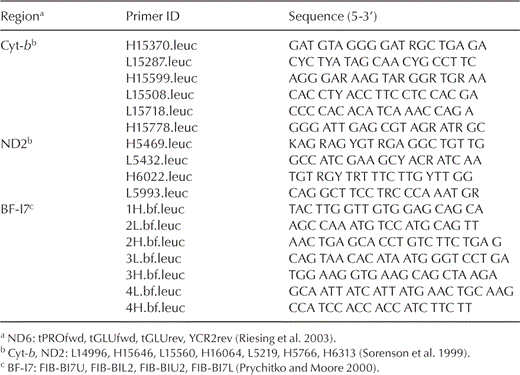
Corrected sequence divergence (csd) estimates among taxa were calculated using Tamura-Nei distances (Tamura and Nei 1993) in MEGA, version 2.1 (Kumar et al. 2001). Empirical base frequencies and nucleotide composition bias were calculated in PAUP* (Swofford 2004). Substitution saturation plots were constructed by codon position and gene for mitochondrial loci in DAMBE using (Xia 2000) Tamura-Nei genetic distances (Tamura and Nei 1993) and pairwise numbers of transitions and transversions.
Phylogenetic reconstruction was done using maximum parsimony (MP), maximum likelihood (ML), and Bayesian inference (BI) separately on each gene or intron and then on multilocus data sets (see below). The MP trees were constructed in PAUP*, version 4.0b10 (Swofford 2004), using heuristic searches with starting trees obtained by random addition of taxa with 10 replicate searches and tree-bisection-reconnection (TBR) branch-swapping for 1,000 bootstrap replicates. Gaps were treated as a fifth state, and missing data were treated as uncertainties.
Nonparametric bootstrap ML analyses were conducted on unpartitioned data sets in GARLI, version 0.94 (Zwickl 2006). GARLI applies a genetic algorithmic approach similar to that of GAML (Lewis 1998). Sequence evolution models are implemented in a manner analogous to that conducted in PAUP* (Swofford 2004), such that resulting log likelihood scores are directly comparable to those that would be recovered in PAUP* analyses of sufficient length. We used MODELTEST, version 3.7 (Posada and Crandall 1998), to determine the best-fit model for each gene, intron, and codon position with the hierarchical likelihood ratio test, all characters equally weighted, and a neighbor-joining starting tree as implemented in PAUP* (Swofford 2004). The simplest model with the lowest Akaike's Information Criterion (AIC) was chosen for analyses. Bootstrap runs for ML analyses consisted of 500 pseudoreplicate heuristic searches with a GTR + I + G model.
Models with similarly low AIC values were applied separately for each gene, codon position, and intron in MRBAYES, version 3.1.2 (Huelsenbeck and Ronquist 2001), using four Markov chains sampling every 500 generations for 6 million generations. For each run, the distributions of parameter sampling were visualized and burn-in periods assessed in TRACER, version 1.1 (see Acknowledgments). Conservative burn-in periods of 10% were sufficient for all runs. In all cases, the resulting topologies were identical, regardless of the model used; therefore, the simplest model producing the most even distribution of sampling with the greatest number of independent samples (effective sample size [ESS] values in TRACER) was chosen for Bayesian inference (Alfaro and Huelsenbeck 2006).
We assessed four partitioning schemes for joint analyses of cyt-b and ND2: one partition including both genes, one partition for each gene (two partitions), one for each codon position (three partitions), and one for each codon position in each gene (six partitions). Similarly, ND6 was assessed as a single partition and as three partitions, each corresponding to a different codon position. Joint analyses with the nuclear intron forming a separate partition from the cyt-b and ND2 data were performed after the best partitioning strategy was determined (see below). Parameters were allowed to vary independently for each partition during MRBAYES runs. Harmonic mean log likelihoods for each partitioning scheme were calculated using the "sump" command in MRBAYES (Table 3). Bayes factors were calculated for each pairwise combination of partitioning schemes as an objective criterion for determining the best partitioning strategy for final analyses (Brandley et al. 2005). Three independent BI analyses using the partitioning strategy with the highest likelihood score were conducted to test for convergence on similar likelihood scores and topologies.
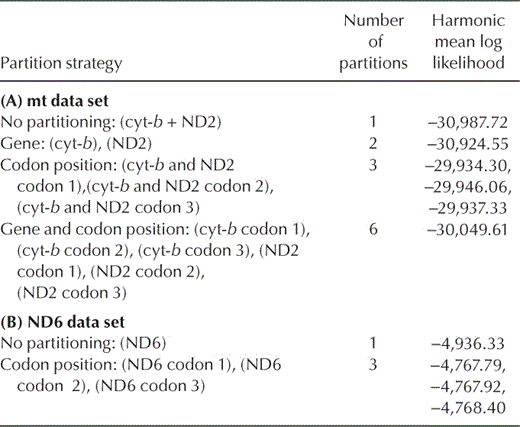
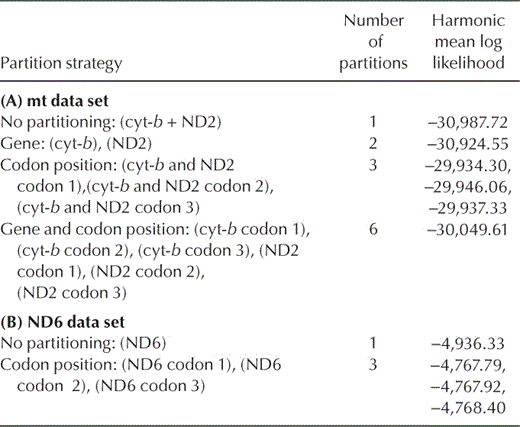
Results and Discussion
Sequence characteristics and phylogeny.—Numbers of parsimony-informative sites and variable but uninformative sites, respectively, were 487 and 48 out of a total 1,120 aligned base pairs (bp) of cyt-b, 564 and 74 out of 1,047 bp of ND2, 122 and 189 out of 981 bp of BF-I7, and 200 and 42 out of 519 bp of ND6. Empirical base frequencies correspond to those found in other avian studies (mitochondria: A, ~30%; C, ~35%; G, ~10%; T ~24%; BF-I7: A = 31%; C = 17%, G = 18%; T = 33%). The chi-square test of homogeneity showed no significant nucleotide composition bias across taxa.
Substitution saturation plots (not shown) show nearly linear increases of both transitions and transversions, with a steeper slope for transitions than for transversions, except for third-base-codon positions in ND6, which show some saturation beginning at a genetic distance of ~7%.
An insertion of three adenines was found in Accipiter nisus and A. rufiventris directly preceding the stop codon of cyt-b. Autapomorphic indels in BF-I7 ranged from 1 to 11 bp in length and were found in 12 species. Parsimony-informative indels were found for Circus aeruginosus and C. ranivorus (9-bp deletion), for C. aeruginosus, C. ranivorus, A. bicolor, A. cirrocephalus, A. cooperii, and A. rufiventris (1-bp deletion), and an insertion was found for five of these species (1 bp not shared by A. cirrocephalus). Leptodon cayanensis, Rostrhamus sociabilis, Geranospiza caerulescens, Leucopternis schistaceus, Harpyhaliaetus solitarius, and all four species in Buteogallus share a 2-bp deletion of TG or GT; all four Buteogallus spp., H. solitarius, and L. schistaceus share a 2-bp deletion. Because of ambiguity in the DNA sequence, we could not determine whether the 2-bp deletion (TG or GT) described above was synapomorphic for all nine sampled individuals, so the two bases were excluded from the analyses for all species. Missing data comprised <10 bp for all individuals, except in cyt-b for three individuals: Butastur indicus (216 missing bases), L. p. princeps (308 missing bases), and Buteogallus aequinoctialis (569 missing bases). No significant difference in topology or likelihood was found between analyses of the mitochondrial data set with and without these sequences; thus, mitochondrial analyses shown here include them. Sequences are available at GenBank (EU583221–EU583379); alignments and published trees can be found at TreeBASE (S2001).
Separate phylogenetic analyses of BF-I7 (not shown) produced a less-resolved tree than the other analyses. The relationships among major accipitrid clades were recovered with high support values (Bayesian posterior probability [BPP] = 1.00–0.97), as were most terminal sister relationships; however, the basal branching pattern within the Buteoninae was not resolved beyond finding three separate clades for the buteonine kites and Geranospiza, the species in Buteogallus sister to L. schistaceus and all other Buteoninae (BPP = 0.99). Also, the position of Buteo (or Rupornis) magnirostris was unresolved. Separate analyses of cyt-b and ND2 also produced trees with several buteonine polytomies: (1) a polytomy of three clades: Busarellus, Geranospiza, L. princeps, L. plumbeus, Buteo (or Percnohierax) leucorrhous, and Parabuteo unicinctus; the kites; and the Buteogallus spp., Harpyhaliaetus spp., and L. schistaceus and L. lacernulatus; and (2) a polytomy of the three remaining buteonine clades (clades diverging after node A in Fig. 1, described below). There were two main differences between the separate ND2 and cyt-b analyses: (1) B. (or R.) magnirostris diverged before all other Buteoninae in the cyt-b analyses (BPP = 1.00) but, in the ND2 analyses, was part of an unresolved polytomy with B. (or P.) leucorrhous, Parabuteo unicinctus, and a clade containing the later-diverging Buteoninae (species diverging after node A in Fig. 1, described below; BPP = 0.98); and (2) in cyt-b analyses, the Butastur spp. were part of a five-way polytomy with Kaupifalco, a clade of goshawks (genera Melierax, Micronisus, and Urotriorchis), a clade of accipiters and harriers (genus Circus), and a clade of sea eagles and buteonines (BPP = 1.00), whereas in the ND2 analyses the Butastur spp. were sister to the Ictinia spp. (BPP = 0.74). Because single-locus analyses produced topologies that were similar overall, we performed joint analyses of cyt-b and ND2 and of cyt-b, ND2, and BF-I7.
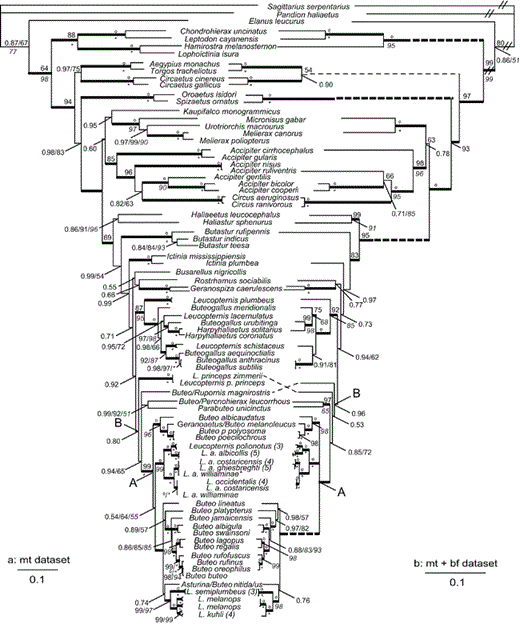
Phylogeny for accipitrid taxa inferred from mitochondrial cyt-b and ND2 (a: mt data set) and nuclear BF-I7 (b: mt + bf data set). Topology shown is the Bayesian-inference majority-rule consensus tree from three independent runs. Bayesian posterior probability (BPP) values of 0.50–0.99 are shown above branches, and values of 1.00 are indicated by a bold line leading to the node. Maximum-likelihood (ML) values are above nodes, following BPP and preceding maximum-parsimony (MP) bootstrap values. Maximum-parsimony bootstrap values >50% are shown in italics below branches or following BPP or ML values. Bootstrap values of 100 are indicated by a circle for ML and by an asterisk for MP. Dashed lines are extensions of branch lengths, and double slash marks indicate branches reduced in length. L. a. williaminae* denotes the type specimen.
Three data sets were assembled. The mitochondrial or "mt" data set included 2,066 aligned (i.e., including indels) base pairs of mitochondrial DNA (1,020 bp cyt-b and 1,046 bp ND2) from 105 individuals of the family Accipitridae, representing 76 named species. The "mt + bf" data set included 3,048 bases of aligned combined mitochondrial and nuclear data (the mt data set appended to 981 bases of BF-I7) for 73 accipitrid taxa representing 56 nominal species. The "ND6" data set included 519 aligned bases of ND6 for 110 taxa representing 47 nominal species.
For the mt data set, the first codon position was modeled by HKY + I + G and the second and third codon positions were modeled by GTR + I + G. The mt + bf data set had four independent partitions: the three mitochondrial partitions described above and a separate partition for BF-I7 using the GTR + G model. For the ND6 data set, the first and third positions were modeled with GTR + G; the second codon position was modeled with HKY + G.
Bayesian consensus trees are shown in Figure 1A for the mt data set, in Figure 1B for the mt + bf data set, and in Figure 2 for the ND6 data set. Posterior probabilities (averages from three independent Bayesian analyses) and MP and ML bootstrap values are shown on the figures. The three different types of analyses produced largely congruent topologies; the few differences involved nodes resolved with low support in the BI analyses and not resolved in the MP or ML analyses. For instance, the branching pattern of the buteonine kites and Geranospiza was unresolved in MP and ML runs and supported by low posterior probabilities in Bayesian analyses (BPP = 0.51–0.77).
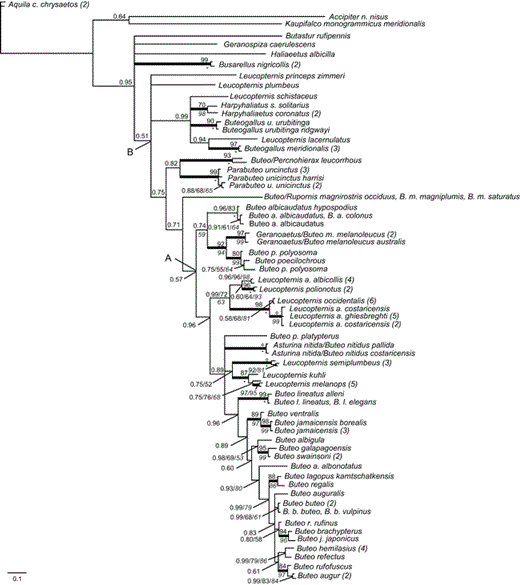
Phylogeny for accipitrid taxa inferred from ND6 sequences. Topology shown is the Bayesian-inference majority-rule consensus tree from three independent runs. Bayesian posterior probability (BPP) values of 0.50–0.99 are shown above branches, and values of 1.00 are indicated by a bold line leading to the node. Maximum-likelihood (ML) values are above nodes, following BPP and preceding maximum-parsimony (MP) bootstrap values. MP bootstrap values >50% are shown in italics below the branches or following BPP or ML values. Bootstrap values of 100 are indicated by a circle for ML and by an asterisk for MP analyses.
Nodes were supported with BPP ≥ 0.90 for 85% of mt and mt + bf nodes. Analyses resolved nearly all nodes in the mt + bf analyses with higher BPP values than with the mt data set alone, perhaps as a result of the larger number of base pairs in the mt + bf data set. For example, the placement of Buteo (or Rupornis) magnirostris and B. lineatus were unresolved in the Bayesian analyses of the mt data set but were resolved in the mt + bf analyses with high support (BPP = 0.96 and 0.98, respectively).
The phylogeny recovered in analyses of the ND6 data set (Fig. 2) largely agrees with the topologies in Figure 1, except for a polytomy of deeper divergences within and directly preceding the Buteoninae (i.e., placement of Haliaeetus, Busarellus, Geranospiza, and Butastur), which likely results from increased substitution saturation for this gene among older divergences. Within the Buteoninae, the positions of L. princeps, L. plumbeus, Buteo p. platypterus, and Asturina nitida–B. nitidus were unresolved. The ND6 analyses differ from the mt analyses in that they recover a sister relationship between L. lacernulatus and Buteogallus meridionalis and show an earlier but unresolved divergence of B. platypterus. This could reflect differences in taxon sampling between the analyses, differences between samples of L. lacernulatus (ND6 sequence from do Amaral et al. 2006), or differences in their molecular evolution, given that ND6 is the only mitochondrial protein-coding gene encoded by the light strand. Our ND6 analyses were concordant with previous studies (Riesing et al. 2003, do Amaral et al. 2006) except that we found a sister relationship between B. r. rufinus (not B. auguralis as in fig. 2 of Riesing et al. [2003]: MP bootstrap = 83, neighbor-joining support = 82) and a clade containing B. brachypterus and B. j. japonicus (BPP = 0.90; Fig. 2). Other differences between our analyses and those of Riesing et al. (2003) involve nodes supported by bootstrap values of <50% in their figures.
Old World taxa (Kaupifalco and Butastur) and Accipiter.—Three species in the genus Butastur form a monophyletic group (BPP = 1.00; Fig. 1A, B) diverging after the sea eagles but before the other sub-buteos, in a clade that is not closely related to Kaupifalco. By including representatives from each previously identified clade or subfamily of Accipitridae and expanding the sampling of harriers, accipiters, and goshawks, we found that Kaupifalco is sister to a clade including Melierax, Micronisus, and Urotriorchis (BPP = 0.95; Fig. 1A) and sister to an Accipiter sp. when the goshawks and other non-Buteonine genera were not included (BPP = 0.64; Fig. 2). Kaupifalco and Butastur, both described as sub-buteos by Amadon (1982), were later removed from the group by Amadon and Bull (1988). Kaupifalco was removed on the basis of observations by Kemp that the "voice and habits" of Kaupifalco are more similar to those of Melierax than to those of sub-buteos (Amadon and Bull 1988). Amadon and Bull (1988) also removed Butastur from Buteoninae, emphasizing its similarity to Kaupifalco. Our results confirm that Kaupifalco is indeed more closely related to Melierax than to sub-buteos but show that Butastur is more closely related to the sub-buteos than to the clade containing Kaupifalco and Melierax. Therefore, of the two Old World genera, we find support only for Butastur as a buteonine genus.
With this expanded sampling, we also found non-monophyly of the genus Accipiter when Circus spp. are included. In the mt data set, two Circus spp. are nested within a clade of seven Accipiter spp. (BPP = 0.82; Fig. 1A) or three accipiters (BPP = 1.00; Fig. 1B). This finding that Circus is nested within the larger Accipiter clade has not been published previously, as far as we know. Earlier studies including both genera, based on smaller sets of taxa and characters with less detailed searches, did not find Accipiter polyphyly but indicated reciprocal monophyly of the genera and a close but non-sister relationship instead (Wink and Seibold 1996, Wink and Sauer-Gurth 2004). Our finding of Accipiter polyphyly is also supported in analyses with greater sampling of species in both genera that are part of a larger consideration of Accipitridae (H. R. L. Lerner et al. unpubl. data).
Black-collared Hawk (Busarellus nigricollis).—Busarellus diverges early within the Buteoninae, after a clade of Butastur spp. and sister to Rostrhamus and Geranospiza, with low support in the mt analyses (BPP = 0.55; Fig. 1A) or unresolved with respect to Butastur, Geranospiza, and Haliaeetus (BPP = 0.95; Fig. 3). Previously proposed sister groups for Busarellus include milvine kites and sea eagles (Ridgway 1876, Olson 1982), sub-buteos Buteogallus and Parabuteo (Brown and Amadon 1968), or Hieraaetus and Polemaetus (Holdaway 1994). We did not find a well-supported close sister relationship for Busarellus here, but we confirmed its position within Buteoninae.
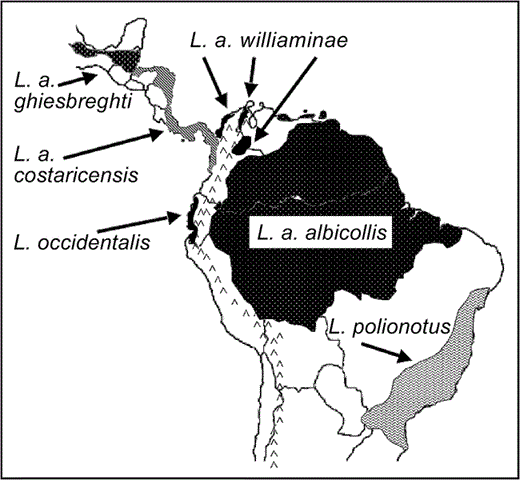
Geographic distribution of White Hawk (Leucopternis albicollis) and related taxa, compiled from published descriptions and maps (Slud 1964, Wetmore 1965, Monroe 1968, Land 1970, Hilty and Brown 1986, Thurber et al. 1987, Sick 1993, Howell and Webb 1995, Ferguson-Lees and Christie 2001, Hilty 2003, Jones 2003, BirdLife International 2004).
Relationships among and within genera of New World Buteoninae.—Divergence of Ictinia follows that of the sea eagles and the genus Butastur (BPP = 0.99, 0.97; Fig. 1). Rostrhamus is sister to Geranospiza but with low support (BPP = 0.66, 0.77; Fig. 1). With nearly complete sampling in Leucopternis and Buteogallus, we confirmed their non-monophyly (Lerner and Mindell 2005, do Amaral et al. 2006). Both L. schistaceus and L. plumbeus had been placed in the genus Urubitinga (Sharpe 1874, Ridgway 1876), now synonymous with Buteogallus (Peters 1931, AOU 1988), on the basis of morphological similarities with B. urubitinga and B. anthracinus. Here, we find that these two Leucopternis spp. are indeed more closely related to Buteogallus than to other Leucopternis spp.; however, they are not sister taxa as proposed by Amadon (1982).
The clade including some Leucopternis spp., all Buteogallus spp., and both Harpyhaliaetus spp. shows a well-supported split between species that are dependent on aquatic habitats such as mangroves, marshes, forest, and wetlands (B. aequinoctialis, B. anthracinus, B. subtilis, and L. schistaceus) and mostly forest or open-vegetation habitats (L. lacernulatus, B. urubitinga, H. solitarius, and H. coronatus; Fig. 1) (BPP = 0.98 and 1.00; Ferguson-Lees and Christie 2001).
Leucopternis spp. are members of four different non-sister clades within the Buteoninae (Fig. 1; two species unresolved in Fig. 2). We found that L. princeps is more closely related to a large clade of Buteo and other Leucopternis taxa (BPP = 0.80 and 0.53, Fig. 1; unresolved in Fig. 2) than to a clade of Buteogallus, Leucopternis, and Harpyhaliaetus (fig. 1 in do Amaral et al. 2006: BPP = 0.68, bootstrap = 58). The lack of resolution for L. princeps in Fig. 2 and the difference between Fig. 1 and the results of do Amaral et al. (2006, their fig. 1) likely reflect differences in the size and informativeness of the data sets.
Genetic divergence among Buteogallus subtilis, B. anthracinus, and B. aequinoctialis.—The individual Mangrove Black Hawks (B. subtilis) and Common Black Hawks (B. anthracinus) we sampled had identical BF-I7 sequence, only a 1-bp difference in cyt-b, and another single difference in ND2, whereas the Rufous Crab-Hawk (B. aequinoctialis) was different from both of these species at 20 mitochondrial bases (2% csd). Buteogallus subtilis has been considered a subspecies of B. anthracinus and a member of a superspecies with B. aequinoctialis (Brown and Amadon 1968). Given that these three taxa are distributed in adjacent and sometimes overlapping ranges in similar habitat on the Atlantic and Pacific coasts and islands of the New World tropics, potential for interbreeding exists, and broader geographic sampling is needed before taxonomic revisions can be made.
Non-monophyly of nominal White Hawk subspecies (L. albicollis).—We sampled two to five (average = 4) individuals from the broad geographic range of each White Hawk subspecies, the Grey-backed Hawk (L. occidentalis) and the Mantled Hawk (L. polionotus; Fig. 3). The White Hawk was not monophyletic, with the nominate form (L. a. albicollis) more closely related to L. polionotus than to other subspecies of L. albicollis (BPP = 1.00, Fig. 1; BPP = 0.60, Fig. 2). Individuals of L. a. albicollis are 2.3% (mt csd) divergent from individuals of L. polionotus, a value similar to that found for other accipitrid sister taxa (Lerner and Mindell 2005: 95–98% sequence similarity for booted eagles; Johnson et al. 2006: 0.5–3.8% csd among Gyps spp.).
The three trans-Andean (i.e., occurring west of the Andean cordillera) subspecies of L. albicollis and L. occidentalis share mt haplotypes (Fig. 1A) and exhibit gradation of plumage coloration from nearly all-white birds in the north (L. a. ghiesbreghti) to heavy black coloration on the heads and wings of southern birds (L. occidentalis; H. R. L. Lerner et al. unpubl. data). Individuals of the most northern subspecies, L. a. ghiesbreghti, formed a clade sister to representatives of L. occidentalis, the most southern species; however, individuals of two White Hawk subspecies occurring in the center of the trans-Andean range for these taxa (L. a. costaricensis and L. a. williaminae from southern Central America and northern South America) were found in both clades. The subspecies L. a. williaminae has a very small range and is known from only a few museum specimens (the type specimen is indicated by an asterisk after the name on Fig. 1). The two clades identified in trans-Andean birds do not strictly correspond to current taxonomy, geography, or plumage coloration. These clades diverge by an average 1.2% (mt csd), which is similar to, but on the low end of, the divergence observed between other accipitrid sister-species pairs (Lerner and Mindell 2005, Johnson et al. 2006). Members of the trans-Andean clades differ from their sister clade containing L. a. albicollis and L. polionotus by 4.4% (average mt csd).
Analyses with greater sampling of individuals are needed, but the current set of relationships based on mitochondrial data (Figs. 1A and 2, but not Fig. 1B) support recognition of L. a. albicollis as L. albicollis and of L. a. costaricensis, L. a. ghiesbreghti, and L. a. williaminae as one or more distinct species. Four to six individuals of the endangered L. occidentalis form a monophyletic (Fig. 2) or unresolved group nested within a clade of individuals of L. a. costaricensis, L. a. ghiesbreghti, and L. williaminae (Fig. 1). None of these clades was recovered with nuclear intron data alone. This may reflect differences in expected coalescence times among maternally versus biparentally inherited loci, especially if these divergences are recent or if the effective population sizes are large (Hudson 1990). Using more variable loci, additional specimens, and population genetic methods could help in further taxonomic assessment and in distinguishing between alternative hypotheses, such as incipient speciation, secondary contact, or isolation by distance, for this clade. Given the status of the small and isolated populations of L. occidentalis, such analyses could be useful for conservation programs.
Genetic divergence between L. kuhli and L. melanops.—White-browed Hawks (L. kuhli) and Black-faced Hawks (L. melanops) are similar in appearance and are considered separate but closely related species (Hellmayr and Conover 1949, Amadon 1982). There were no shared mt or BF-I7 haplotypes between the species, and with mt data they are 1.8% divergent from each other. The polytomy in Figure 1A, however, precludes strong conclusions in this regard. The four individuals of L. melanops are nearly as divergent from each other as they are from individuals of L. kuhli, with 1.4% average csd, whereas the average csd among four conspecific individuals of L. kuhli is 0.56%. Using the more variable ND6 data set plus additional pseudo-control-region sequence, two individuals of L. melanops from Peru are 0.24% divergent from each other and, on average, 2.04% divergent from a Peruvian L. kuhli. These values are similar to, but on the low end of, those found between other closely related accipitrid species (see above).
Although the two species were originally described as allopatric, potential for hybridization exists, given that individuals of L. melanops have been trapped simultaneously with L. kuhli south of the Amazon river (Olalla collections of 1930 at the American Museum of Natural History [AMNH], and recent trappings described in Barlow et al. 2002). The two species, however, appear to be identifiable by plumage: about 20 specimens of each species examined at the AMNH were distinct in plumage, with no intermediate plumage types observed. Given the high level of genetic diversity within L. melanops, the lack of resolution of the mitochondrial data set, and the potential for hybridization, further analysis of these two species or this "superspecies" is warranted.
Phylogeny and taxonomy of the genus Buteo.—In Figure 1, all members of the nominal genus Buteo diverge after the node labeled "B." Following the early divergence of L. princeps and B. (R.) magnirostris, a sister relationship between B. (Percnohierax) leucorrhous and Parabuteo unicinctus is supported (BPP = 0.99 and 1.00, Fig. 1; BPP = 0.82, Fig. 2). The remaining Buteo species fall into two clades: (1) B. albicaudatus, Geranoaetus melanoleucus, B. poecilochrous, and B. polyosoma and (2) all others (11 species in Fig. 1 and 18 species in Fig. 2). The positions of B. lineatus, Asturina nitida–Buteo nitidus, and B. jamaicensis have not been resolved or well supported previously (nodes III [MP bootstrap = 58, neighbor-joining support = 90] and IV [support values <50] in Riesing et al. 2003). In Figure 1, we find that the divergence of B. lineatus (BPP = 0.98, Fig. 1B) is followed by that of B. platypterus (BPP = 0.97, Fig. 2B), and Asturina nitida–B. nitidus is more closely related to several species in the genus Leucopternis than to these two Buteo spp. (BPP = 0.74 and 0.76, Fig. 1; node III in Riesing et al. 2003). We also find that divergence of B. jamaicensis (BPP = 1.00, Fig. 1) is followed by divergence of the sister species B. albigula and B. swainsonii (BPP = 1.00 and 0.88, Fig. 1; BPP = 0.65, Fig. 2). Within the Buteoninae, we find that earlier divergences correspond to taxa with New World distributions followed by the sister pair of Nearctic B. regalis and circumpolar B. lagopus (BPP = 1.00, Figs. 1 and 2) and all Old World taxa diverging last (Figs. 1 and 2; see also Riesing et al. 2003).
We support the idea that taxonomy should reflect phylogeny. In that spirit, one proposal for redefinition of the genus Buteo includes all species descended from node A (Figs. 1 and 2, and Riesing et al. 2003). With the data set used by Riesing et al. (2003), this proposal would have required changing the generic names of three species (Asturina nitida to Buteo nitidus, B. magnirostris to Rupornis magnirostris, and B. leucorrhous to Percnohierax leucorrhous). Delimiting the genus Buteo at node A of Fig. 1 in our analyses would require changing the generic names of an additional six Leucopternis spp. as well as the genus Geranoaetus. We recommend delimiting Buteo earlier in the tree at node B (Fig. 1), so that it comprises a single clade including all current members of the genus Buteo sampled in both studies; this involves a change in genus name for two more species (Parabuteo unicinctus and L. princeps).
Acknowledgments
Our sincere thanks to specimen collectors, curators, and museum staff for providing samples (see Table 1), and we particularly thank D. Whitacre, N. Rice, M. Watson, D. Susanibar, J. Antonio, O. Beingolea, A. V. Benavides, N. Benavides, R. Luna, and J. Hendriks. R. B. Payne, D. Lahti, N. David, and A. Peterson clarified nomenclatural issues. J. Johnson, M. Bunce, M. Lerner and J. Brown provided valuable expertise on laboratory techniques or data analysis. R. B. Payne, C. Dick, L. Kiff, two anonymous reviewers, and R. Brumfield provided helpful comments on the manuscript. This research was supported by grants from the University of Michigan (UM) Museum of Zoology; UM Department of Ecology and Evolutionary Biology; UM Rackham Graduate School; UM Latin American and Caribbean Studies Program; National Institutes of Health (support for H.R.L.L.); UM College of Literature, Science and the Arts; Whittaker Grant for Undergraduate Education (support for M.K.); and the National Science Foundation (support for D.M.). GARLI software is available at www.bio.utexas.edu/faculty/antisense/garli/Garli.html. TRACER software is available at tree.bio.ed.ac.uk/software/tracer/. A study by Griffiths et al. (2007) was published after acceptance of the current paper and should be consulted for accipitrid phylogenetic analyses based on a nuclear RAG-1 exon.
Literature Cited
Author notes
Present address: National Zoological Park, Smithsonian Institution, Genetics Lab, 3001 Connecticut Avenue, NW, Washington, D.C. 20008, USA. E-mail: [email protected]
Present address: California Academy of Sciences, 55 Concourse Drive, Golden Gate Park, San Francisco, California 94118.
Associate Editor: R. T. Brumfield