-
PDF
- Split View
-
Views
-
Cite
Cite
Andrew C. Vitz, Amanda D. Rodewald, Vegetative and Fruit Resources as Determinants of Habitat use by Mature-Forest Birds During the Postbreeding Period, The Auk, Volume 124, Issue 2, 1 April 2007, Pages 494–507, https://doi.org/10.1093/auk/124.2.494
- Share Icon Share
Abstract
Recent studies have demonstrated that many birds of mature forests heavily use early-successional habitat during the postbreeding period. Two frequently invoked hypotheses to explain these shifts are that postbreeding birds select (1) dense cover to reduce risk of predation and (2) abundant fruit resources to facilitate foraging. Using mist nets between 15 June and 16 August in 2002 and 2003, we captured mature-forest birds during the postbreeding period in 12 regenerating hardwood clearcuts (three to seven years old) in southeast Ohio. Vegetation structure and fruit resources were measured at nine net locations within each clearcut. We applied an information-theoretic approach, where we used vegetative and fruit variables as predictors in seven a priori models to evaluate how habitat factors might explain capture rates. In two seasons, we captured 1,089 hatch-year (HY) and 445 after-hatch-year (AHY) postbreeding birds of 32 mature-forest species. In general, models reflecting habitat structure (density of low vegetation, canopy height) best explained variation in capture rates, which were negatively related to the density of low vegetation (<1.5 m) and positively related to canopy height. Extremely dense low vegetation may be disadvantageous if it inhibits movements and provides cryptic locations for ground predators. Instead, habitats with greater vertical structure (e.g., taller vegetation) and relatively less dense low vegetation below may provide better protection from aerial and ground predators. Overall, results suggest that vegetation structure may explain high use of early-successional forests by many birds during the postbreeding period, though fruit may be the most important factor for seasonal frugivores.
Recursos Vegetativos y de Frutos como Determinantes del Uso de Hábitat por Aves de Bosque Maduro Durante el Período Posterior a la Reproducción
Resumen
Varios estudios han demostrado que muchas aves de bosque maduro utilizan hábitats en estados sucesionales tempranos intensamente después del período reproductivo. Las dos hipótesis que pueden explicar estos cambios son: (1) que las aves post-reproductivas seleccionan áreas con una cobertura densa para disminuir el riesgo de depredación y (2) que las aves post-reproductivas seleccionan áreas con abundancia de frutos para facilitar el forrajeo. Utilizando redes de niebla entre el 15 de junio y el 16 de agosto de 2002 y 2003, capturamos aves de bosque maduro durante el periodo post-reproductivo en 12 bosques en regeneración posterior a tala rasa (de tres a siete años de edad) en el sudeste de Ohio. Medimos la estructura de la vegetación y la abundancia de frutos en nueve localidades de captura de aves en cada bosque secundario. Aplicamos un enfoque de teoría de la información en el cual utilizamos variables vegetativas y de frutos como variables de predicción en siete modelos definidos a priori para determinar cómo los factores de hábitat podrían explicar las tasas de captura. Durante dos estaciones, capturamos 1,089 individuos nacidos en el año de muestreo y 445 individuos post-reproductivos nacidos en años anteriores pertenecientes a 32 especies de bosque maduro. En general, los modelos que incluyeron variables de estructura del hábitat (densidad de la vegetación baja, altura del dosel) fueron los mejores para explicar la variación en las tasas de captura, las cuales estuvieron relacionadas negativamente con la densidad de la vegetación baja (<1.5 m) y positivamente con la altura del dosel. Una vegetación baja extremadamente densa puede ser desventajosa si inhibe el movimiento y si provee lugares crípticos para depredadores terrestres. Contrariamente, los hábitats con una mayor estructura vertical (e.g., vegetación más alta) y con una vegetación baja relativamente menos densa pueden proveer de una mejor protección ante depredadores aéreos y terrestres. En general, estos resultados sugieren que la estructura de la vegetación puede explicar el uso intenso de bosques en estados sucesionales tempranos durante el período post-reproductivo, a pesar de que la presencia de frutos puede ser el factor más importante para los frugívoros estacionales.
Population declines have plagued several mature-forest species throughout eastern North America (Robbins et al. 1989, Terborgh 1989, Askins 2001), and these declines have stimulated much research on habitat loss and fragmentation on both the breeding and wintering grounds (Terborgh 1989, Askins et al. 1990). However, little attention has been given to the postbreeding period, which is defined as following nesting or fledging and continuing until the onset of fall migration, lasting up to three months (Pagen et al. 2000). This may be a particularly hazardous time for juveniles, because they are inexperienced at both foraging (Breitwisch et al. 1987, Desrochers 1992, VanderWerf 1994) and detecting and evading predators (Sullivan 1989, Wunderle 1991). Adults may be vulnerable because of inhibited flight resulting from their prebasic molt (Vega Rivera et al. 1998a). Indeed, previous work demonstrates that mortality rates can be extremely high for juveniles during the post- breeding period (Krementz et al. 1989, Sullivan 1989, Anders et al. 1997), which may profoundly affect population demography (Anders et al. 1997). Consequently, unless a more thorough understanding of avian postbreeding habitat requirements is achieved, conservation strategies may overlook important seasonal needs and unintentionally compromise survival.
Although habitat requirements of mature- forest birds have traditionally been assumed to be similar across much of their annual cycle, habitat-use patterns can shift dramatically over the seasons (Moore et al. 1990, Vega Rivera et al. 1999, Pagen et al. 2000). For example, mature-forest birds often use early-successional habitat at migratory stopover sites (Moore et al. 1990, Rodewald and Brittingham 2004) and on their wintering grounds (Petit et al. 1995), which may result from seasonal frugivory (Parrish 2000). Moore et al. (1990) documented the greatest abundance and diversity of migrating birds in shrub-scrub habitat despite it representing only 14% of available habitat, which suggests that birds actively selected this habitat (Johnson 1980). On the wintering grounds, numerous studies have documented greater abundance of Neotropical migrants (including mature-forest breeders) using shrubby rather than forest habitats (Karr 1976, Terborgh 1980). Similarly, research on mature-forest birds during the post- breeding period, albeit limited, has revealed that young and adults of a number of species heavily use early-successional forests (Anders et al. 1998; Vega Rivera et al. 1998b, 1999; Pagen et al. 2000; Vitz and Rodewald 2006). This shift in habitat use may reflect habitat selection based on probability of survival, because birds are no longer constrained by breeding requirements.
A common suggestion for explaining this shift in habitat use is that juveniles and post- breeding adults seek out early-successional habitat partly for cover and food resources (Desrochers 1992). The dense vegetation and abundant fruit resources generally found in early-successional forests may be especially important for survival of birds during the postbreeding period. Juveniles are extremely vulnerable to predators because of naiveté and poor flight skills (Sullivan 1989, Anders et al. 1997), and adults also may be vulnerable because of compromised flight while they undergo their prebasic molt. Furthermore, numerous studies have documented lower foraging proficiency by juveniles compared with adults (Desrochers 1992, VanderWerf 1994), and this lack of foraging proficiency may result in starvation (Krementz et al. 1989, Sullivan 1989). Juveniles may compensate for their lack of foraging skill by concentrating on food items that are easily captured or found (Breitwisch et al. 1984, Stevens 1985). Fruit not only provides an easily captured and abundant food resource (Snow 1971) but may attract insects, further enhancing foraging opportunities. Abundant food resources also decrease the need to move widely in search of food, reducing energy loss and exposure to predators. Fruits having high sugar content are especially good food resources for accumulating fat reserves to facilitate migration (Parrish 2000). At the same time, though, dense cover that provides protection from predators may be the primary impetus for selecting early-successional habitats during the postbreeding period. Regenerating clearcuts have leaf area comparable to that of mature forest, all compressed into a few meters above the ground (Keller et al. 2003), providing protective cover. We explicitly compared the relative importance of food versus structural resources for individuals during the postbreeding period.
Methods
Study area.—
The study area was in southeast Ohio, within the Zaleski State Forest and Mead- Westvaco forest lands (Athens, Vinton, Gallia, and Jackson counties). The area is characterized by rolling hills and is located within the Ohio Hills physiographic province, which is ∼70% forested. For a description of the study area and a list of common breeding birds and tree species, see Vitz and Rodewald (2006).
We studied birds during the postbreeding period at 8 regenerating clearcut sites in 2002 and 12 sites in 2003 (adding 4 new sites to the original 8). Sites ranged from three to seven years postharvest, were 4–18 ha in size, and were separated by ≥1 km. Because regenerating clearcuts comprise dense vegetation, providing limited visibility, sight-based surveys are difficult to conduct. This difficulty is exacerbated during the postbreeding period, when songbirds are notably quiet and furtive (Pagen et al. 2000). Therefore, constant-effort mist netting was done between 15 June and 16 August each year. Mist nets avoid problems associated with visual and auditory detection and better sample secretive or rarely vocal species (Karr 1981). Because mist nets sampled nearly the entire vegetative structure of our regenerating clearcuts, we were able to minimize bias associated with this method (Remsen and Good 1996).
Net placement.—
We used nine nets (12 × 2.6 m, 30-mm mesh) per site, and two sites were sampled simultaneously each day. Sites were sampled approximately once per week, with a total of nine visits per year. Nets were arranged systematically at each site, and three parallel net transects were separated by 50 m and extended perpendicularly from a common mature-forest edge. Within each transect, nets were placed 20, 50, and 80 m from the mature- forest edge, parallel to the edge, the distance of any portion of the net to another mature-forest edge being ≥80 m (except in a few cases where the edge was 70 m away). Nets were opened half an hour before sunrise, closed 4.5 h later, and cleared of birds every 30 min. Nets were moved between sites daily to reduce net avoidance by birds. All birds captured, except Ruby- throated Hummingbird (Archilochus colubris), were banded with a federal band, and information collected included species, age, sex, molt, mass, fat, wing chord, and breeding condition. Birds captured during the postbreeding period included all hatch-year (HY) individuals and after-hatch-year (AHY) birds showing a wrinkled brood patch, flight-feather molt, or extensive body molt (>25% of body), indicating completion of nesting (Pyle 1997). Although some individuals of certain species are known to occasionally overlap breeding and molting, these stages seldom coincide, because of energy constraints (Murphy and King 1992, Butler et al. 2002; but see Norris et al. 2004). Further evidence that mature-forest birds were not breeding in (or even using) the regenerating stands was provided by the low number of detections of these individuals during breeding surveys (point counts) and the large majority of captured individuals (>85%) exhibiting post- breeding (rather than breeding) characteristics. Of the nearly 15% of birds lacking postbreeding characteristics, most also lacked breeding characteristics (i.e., brood patch or cloacal protuberance) and were probably floaters.
Fruit sampling.—
Fruits were sampled three times at biweekly intervals from 7 July to 15 August, 2002 and 2003. We purposely initiated fruit sampling at this time because (1) it coincided with the period of greatest use by juveniles and postbreeding adults and (2) most fruits were not ripe before this time. Sampling was conducted within two transects (1 × 12 m each) oriented parallel to and 1 m from both sides of each net (24 m2 per net site; Levey 1988). For each plant species, we recorded total number of fruits among all individual plants within the belt transect. Ripe, unripe, and spent fruits were assessed separately. Fruits that were green to pinkish in color were identified as unripe, dark pink to black fruits were ripe, and desiccated fruits were classified as spent. In the analyses, we used all potential fruit (ripe plus unripe), because it better reflected present and near-future fruit availability (Blake et al. 1990) and was strongly correlated with ripe fruit (r = 0.83, n = 108). This decision was important, because Rubus spp., the dominant fruiting plant (92% of all fruit), both ripens and is consumed quickly, and large quantities of fruit would have been missed if only ripe fruit were examined. Spent fruit were not included in the analysis, because they do not contain much nutritional value (Stiles 1980).
Vegetation sampling.—
Vegetation data were collected within a 0.04-ha circular plot established at the center of each mist net (modified from James and Shugart 1970). Within this circular plot, vegetation structure was measured every 2 m along two 20-m transects at each net (one was established perpendicular to the center of the net, and the other was parallel to and 2 m away from the net) by measuring canopy height and density of low (0.5–1.5 m) and high (1.5– 3.0 m) vegetation. Vegetation density was quantified by recording the number of vegetation hits (i.e., number of times leaves or stems touched a pole) from 0.5–3.0 m in 0.5-m increments using a telescoping pole. Canopy cover (cover >5 m) was assessed every 2 m along each 20-m transect by recording the presence or absence of vegetation at the crosshairs of an ocular tube (0 = no vegetation, 1 = vegetation) and then averaged to generate an estimate of percentages of canopy cover. Within the 0.04-ha plot, the three dominant sapling and shrub species were identified and the numbers of small (12–23 cm diameter at breast height [DBH]), medium (23–38 cm DBH) and large (>38 cm DBH) snags, residual trees, and logs (longer than 1.0 m, with a diameter >7.5 cm) were counted.
Data analysis.—
Before analysis, capture rates at the eight sites sampled in both years were averaged because they did not seem to differ by year. Variables not meeting the assumption of normality were square-root transformed. In the few cases where normality was not completely achieved, we relied on the robustness of balanced designs to use a nested analysis (Quinn and Keough 2003). Because residual trees and snags were uncommon at all sites, they were not included in the analysis. Density of high vegetation was removed from analysis, because it was highly correlated with average canopy height (r = 0.71, n = 108), which had the advantage of characterizing the forest above 3 m.
Vegetation structure of regenerating clearcuts is typically heterogeneous, with patches of saplings interspersed with shrubs and bare ground, and this was true for our sites (Table 1). Consequently, much of the relevant variation in microhabitat features and avian captures occurred at a fine scale. To account for this, we used net-level data (rather than site-averaged) in hierarchical linear models with a nested design. Hierarchical linear models allow simultaneous analysis at multiple nested spatial or temporal levels (Osborne 2000). We used a mixed model where random effects can be specified for both continuous and categorical variables (Littell et al. 2002). Explanatory variables measured at nets were nested within sites. We developed a set of seven a priori candidate models, based on evidence from previous studies, to explain patterns of habitat use of birds during the postbreeding period. An information-theoretic approach was used to build and subsequently rank models containing food (fruit abundance) and cover (density of low vegetation, average canopy height) variables. Because several of these variables may contribute to habitat quality during the postbreeding period, models included combinations of the variables fruit, density of low vegetation, and average canopy height for each of the three groups (all HY and AHY postbreeding mature-forest birds, AHY postbreeders, HY) and six species of interest (Red-eyed Vireo, Wood Thrush, Worm-eating Warbler, Ovenbird, Hooded Warbler, Scarlet Tanager; see Table 2 for scientific names). We calculated delta AIC (Δi, Akaike's Information Criterion) and AIC weights (wi) from AICc values (used for small sample size; Burnham and Anderson 2002) generated using a nested analysis in Proc Mixed (SAS Institute 1990). The model with the smallest AICc was considered the best explanatory model; however, other models with Δi < 2 were considered equally plausible in light of the data (Burnham and Anderson 2002).
Numbers of captures of mature-forest birds in 12 regenerating clearcuts in southeastern Ohio during the postbreeding period, 2002 and 2003.
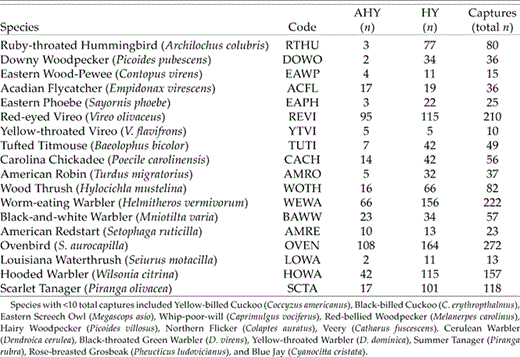
Numbers of captures of mature-forest birds in 12 regenerating clearcuts in southeastern Ohio during the postbreeding period, 2002 and 2003.
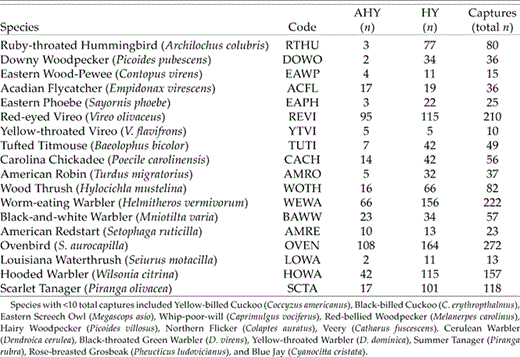
Mean (±SE), range, and coefficient of variation (CV) for habitat variables measured in regenerating clearcuts in southeastern Ohio, 2002 and 2003.
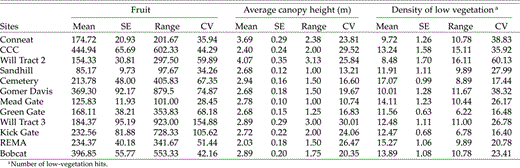
Mean (±SE), range, and coefficient of variation (CV) for habitat variables measured in regenerating clearcuts in southeastern Ohio, 2002 and 2003.
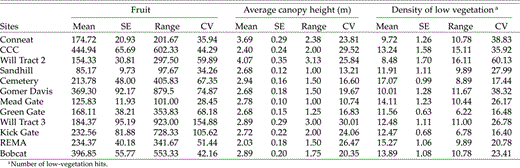
Results
We captured 1,534 mature-forest birds during the postbreeding periods of 2002 and 2003 (only original captures were used in the data set). The total number of mature-forest birds during the postbreeding period consisted of 1,089 HY and 445 AHY individuals of 32 species. The most commonly captured species were Ovenbird, Worm-eating Warbler, Red-eyed Vireo, Hooded Warbler, Scarlet Tanager, and Wood Thrush (Table 2). The lowest capture rates of HY and AHY postbreeders consistently occurred during the first mist-netting visit (mid- to late June), when many individuals were still breeding (Fig. 1A). However, capture rates of mature- forest birds during the postbreeding period quickly increased as nests fledged in adjacent forests (A. Vitz and A. Rodewald unpubl. data). In general, capture rates dramatically increased by 1 July (approximately visit 3) and, for most species, remained high for the duration of the postbreeding season. Two notable exceptions were Scarlet Tanager and Wood Thrush, which peaked in mid-July (visits 5–6) and quickly decreased on subsequent visits (Fig. 1B).
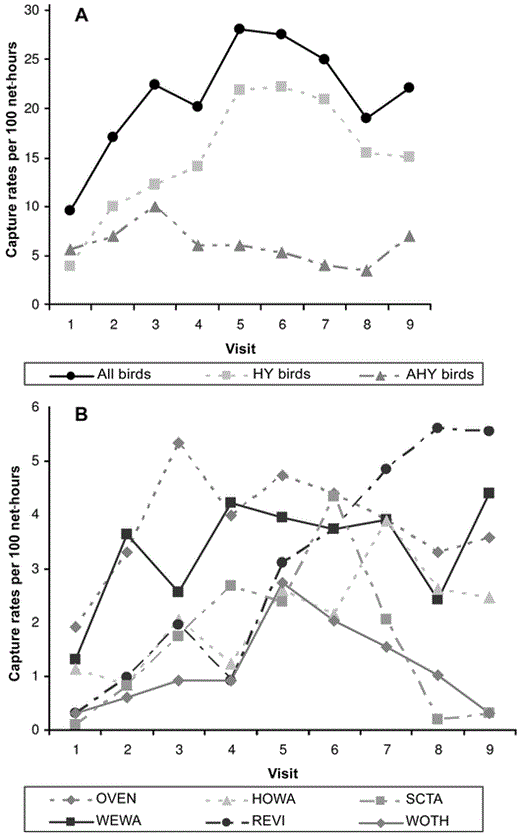
Relationship between captures (per 100 net-hours) and visits for (A) all mature-forest birds during the postbreeding period (All birds), HY birds, and postbreeding AHY birds (AHY birds) and (B) for Ovenbird (OVEN), Worm-eating Warbler (WEWA), Hooded Warbler (HOWA), Red-eyed Vireo (REVI), Scarlet Tanager (SCTA), and Wood Thrush (WOTH) in clearcuts in southeastern Ohio, 2002 and 2003.
Interestingly, capture rates of HY and AHY postbreeders were nearly always negatively related to low vegetation (Fig. 2). Of the seven candidate models, capture rates of all mature- forest birds during the postbreeding period were best explained by the model containing only low vegetation, and this was ranked ∼3× better than the second-best model (Table 3). Similarly, low vegetation best explained captures of HY and AHY birds, for which it was 2.2× and 1.4× more plausible, respectively, than the second-best model (which contained both low vegetation and average canopy height; ΔAIC < 2). The model containing low vegetation was the top model for Worm-eating Warbler and Red-eyed Vireo (Table 3). Worm-eating Warbler was negatively related to low vegetation, whereas Red-eyed Vireo showed a positive relationship. Furthermore, collective weights of evidence (i.e., summed weights for models containing the variable) for low vegetation ranged between 88% and 97%, which suggests that it was an important variable affecting HY and AHY postbreeders. In most cases, models containing both low vegetation and canopy height were considered equally plausible in light of the data (ΔAIC < 2).
Model statements describing microhabitat features in relation to group (all mature-forest postbreeding birds, hatch-year [HY] birds, and after-hatch-year [AHY] birds) and species capture rates in regenerating clearcuts in southeastern Ohio, 2002 and 2003. Best-supported models have a smaller delta AIC (Δi), and larger AIC weights (ωi).
![Model statements describing microhabitat features in relation to group (all mature-forest postbreeding birds, hatch-year [HY] birds, and after-hatch-year [AHY] birds) and species capture rates in regenerating clearcuts in southeastern Ohio, 2002 and 2003. Best-supported models have a smaller delta AIC (Δi), and larger AIC weights (ωi).](https://oup.silverchair-cdn.com/oup/backfile/Content_public/Journal/auk/124/2/10.1093_auk_124.2.494/1/m_i0004-8038-124-2-494-t301.gif?Expires=1750665258&Signature=d7VfsiX3eJuyx8KazcgzezXQUAnEwftNdCkWprjj4zlOCJzXrLot1z-o6Y4pGK1tPQVy0pkj2PdsKgjSTSOpOYTmTkOxaycXaY2lk5aBQVuDKgO2KvHxBCEUxP~dveVebpF98dWgLHTCXXO3CVubLYUDMjqTGQjBY8HjlVRiqqC7PJ~ZeRFQMAsQPwCd6z~aUWoPEO276smdKYSCpdY-O09g6L0Gq3SxKmG5VwNw2bUC6Lru4t~AArSgpJvyNgm-Gz2qj9AxXfrbwlQtQCQnWxm1mkaiFYNz~jUE7jXyz7SqsUQOtzrIVmcfd~1gls3W~x6G4c5APX9~At-eHTKpLA__&Key-Pair-Id=APKAIE5G5CRDK6RD3PGA)
![Model statements describing microhabitat features in relation to group (all mature-forest postbreeding birds, hatch-year [HY] birds, and after-hatch-year [AHY] birds) and species capture rates in regenerating clearcuts in southeastern Ohio, 2002 and 2003. Best-supported models have a smaller delta AIC (Δi), and larger AIC weights (ωi).](https://oup.silverchair-cdn.com/oup/backfile/Content_public/Journal/auk/124/2/10.1093_auk_124.2.494/1/m_i0004-8038-124-2-494-t302.gif?Expires=1750665258&Signature=0h~KyHeaiGlsGavSn9XGHkMQmk2ShhF~54xt9SFYspjqhnKke5I1zif5rqjnis917Epjc~IRDM6WAV80C7~7t7nddntK~S2O50z3uhH7aPsuoZEUW6eIcOIdwxbKu0uh8wijP8~6OQ0m9megsPGOuJQl3EQGwwjG8fqZ6pFxhaHu98kfp8rmfZnUggvwsVl-U1Ct~R~6wnMN6-guOFYKidx~CTMJjVYpaUhQWCgipKqzYgFBj6NAt9~MPXl3H9Y6wNIV2yP19koOrSCS9WdxFf0dUl8pv0-io~F-HKLnu~aaq3EiYgIjBLV8qXohqYaK3RO-SEFRO-YngWmERyxQtw__&Key-Pair-Id=APKAIE5G5CRDK6RD3PGA)
Model statements describing microhabitat features in relation to group (all mature-forest postbreeding birds, hatch-year [HY] birds, and after-hatch-year [AHY] birds) and species capture rates in regenerating clearcuts in southeastern Ohio, 2002 and 2003. Best-supported models have a smaller delta AIC (Δi), and larger AIC weights (ωi).
![Model statements describing microhabitat features in relation to group (all mature-forest postbreeding birds, hatch-year [HY] birds, and after-hatch-year [AHY] birds) and species capture rates in regenerating clearcuts in southeastern Ohio, 2002 and 2003. Best-supported models have a smaller delta AIC (Δi), and larger AIC weights (ωi).](https://oup.silverchair-cdn.com/oup/backfile/Content_public/Journal/auk/124/2/10.1093_auk_124.2.494/1/m_i0004-8038-124-2-494-t301.gif?Expires=1750665258&Signature=d7VfsiX3eJuyx8KazcgzezXQUAnEwftNdCkWprjj4zlOCJzXrLot1z-o6Y4pGK1tPQVy0pkj2PdsKgjSTSOpOYTmTkOxaycXaY2lk5aBQVuDKgO2KvHxBCEUxP~dveVebpF98dWgLHTCXXO3CVubLYUDMjqTGQjBY8HjlVRiqqC7PJ~ZeRFQMAsQPwCd6z~aUWoPEO276smdKYSCpdY-O09g6L0Gq3SxKmG5VwNw2bUC6Lru4t~AArSgpJvyNgm-Gz2qj9AxXfrbwlQtQCQnWxm1mkaiFYNz~jUE7jXyz7SqsUQOtzrIVmcfd~1gls3W~x6G4c5APX9~At-eHTKpLA__&Key-Pair-Id=APKAIE5G5CRDK6RD3PGA)
![Model statements describing microhabitat features in relation to group (all mature-forest postbreeding birds, hatch-year [HY] birds, and after-hatch-year [AHY] birds) and species capture rates in regenerating clearcuts in southeastern Ohio, 2002 and 2003. Best-supported models have a smaller delta AIC (Δi), and larger AIC weights (ωi).](https://oup.silverchair-cdn.com/oup/backfile/Content_public/Journal/auk/124/2/10.1093_auk_124.2.494/1/m_i0004-8038-124-2-494-t302.gif?Expires=1750665258&Signature=0h~KyHeaiGlsGavSn9XGHkMQmk2ShhF~54xt9SFYspjqhnKke5I1zif5rqjnis917Epjc~IRDM6WAV80C7~7t7nddntK~S2O50z3uhH7aPsuoZEUW6eIcOIdwxbKu0uh8wijP8~6OQ0m9megsPGOuJQl3EQGwwjG8fqZ6pFxhaHu98kfp8rmfZnUggvwsVl-U1Ct~R~6wnMN6-guOFYKidx~CTMJjVYpaUhQWCgipKqzYgFBj6NAt9~MPXl3H9Y6wNIV2yP19koOrSCS9WdxFf0dUl8pv0-io~F-HKLnu~aaq3EiYgIjBLV8qXohqYaK3RO-SEFRO-YngWmERyxQtw__&Key-Pair-Id=APKAIE5G5CRDK6RD3PGA)
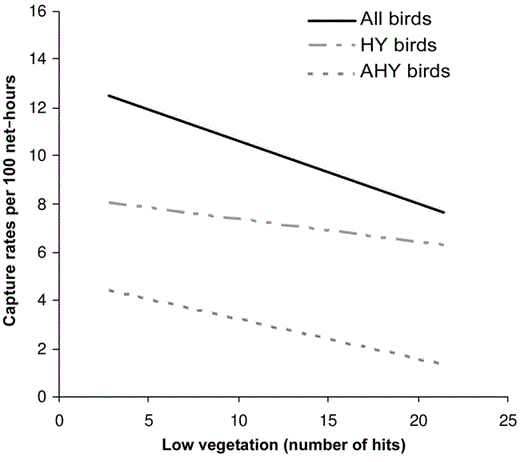
Relationship between density of low vegetation (number of vegetation hits per 0.5–1.5 m) and captures (per 100 net-hours) of all mature-forest postbreeding birds (All birds: Y = −0.2587x), HY (Y = −0.0937x + 8.3298), and postbreeding AHY birds (AHY birds, Y = −0.165x + 4.8746) in southeastern Ohio, 2002 and 2003.
The most consistent top variable across the six species of interest was canopy height, which was positively related to capture rates. The model containing only canopy height was identified as the best model for Ovenbird, Hooded Warbler, and Wood Thrush (Table 3 and Fig. 3). In these cases, the collective weights of evidence for canopy height ranged from 76% to 98%, emphasizing its importance for these species. For Ovenbird, the model containing both fruit and low vegetation was equally plausible. The model containing fruit best explained capture rates for Scarlet Tanager, and fruit abundance was positively related to capture rates of Scarlet Tanager (Fig. 4). The collective weight of evidence for fruit was 99%, providing strong support for its importance (Table 3).
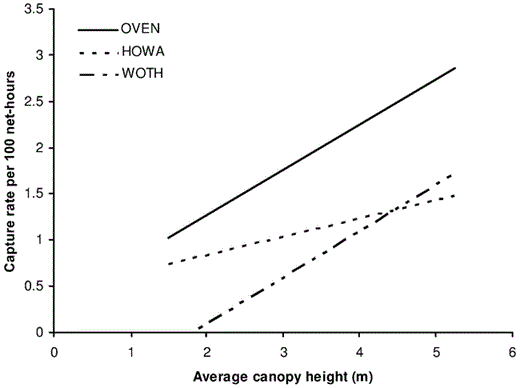
Captures (per 100 net-hours) compared with canopy height for Ovenbird (Y = 0.4895x + 0.2914), Hooded Warbler (Y = 0.1956x + 0.4528), and Wood Thrush (Y = 0.4995x − 0.9033) in southeastern Ohio, 2002 and 2003.
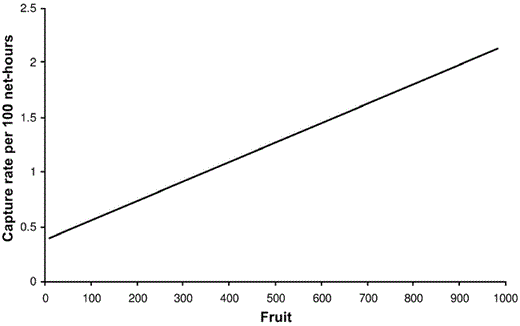
Captures (per 100 net-hours) of Scarlet Tanagers compared with average fruit per net (Y = 0.0018x + 0.3789) in southeastern Ohio, 2002 and 2003.
Discussion
Our results suggest that birds during the post- breeding period seek out structurally complex habitats, as evidenced by the negative associations with density of low vegetation and positive associations with average canopy height. Moreover, these vegetative resources seem to be more important predictors of habitat use than abundant fruit resources. Several studies documenting use of early-successional forests by mature-forest birds during the postbreeding period have suggested that dense cover or abundant food resources, or both, explain observed patterns in habitat use (Anders et al. 1998, Vega Rivera et al. 1998b, Marshall et al. 2003). However, few have explicitly studied the underlying reasons for this shift (but see Vega Rivera et al. 1998b). Our study differs, in that we not only documented substantial use of regenerating clearcuts by mature-forest birds during the postbreeding period, but also evaluated which of two potential causes of this habitat shift have the most support.
Vegetation structure was the most important factor associated with postbreeding bird use of regenerating clearcuts in our study, and this is consistent with suggestions by others (Vega Rivera et al. 1998b, Bayne and Hobson 2001). Although thick vegetation composed of shrubs and saplings characterizes all the regenerating clearcuts we studied, notable spatial heterogeneity persists in this cover, particularly in the lower shrub layer, which can range from impenetrable to relatively open. Interestingly, we recorded higher capture rates in these more heterogeneous areas with more open structure <1.5 m (low vegetation). For example, in these more open areas (<50 vegetation hits), we captured 1.5× and 2.5× the number of HY and AHY postbreeders, respectively, captured in nets surrounded by denser low vegetation (>150 vegetation hits). Extremely dense patches of low vegetation may both inhibit foraging movements by birds and provide cryptic locations for snakes, which are often abundant in early-successional habitats (Thompson and Burhans 2003).
Although our capture data strongly suggest that birds discriminated among regenerating clearcuts on the basis of the density of low vegetation, we cannot dismiss the possibility that these birds selected microhabitats on the basis of shrub canopy height or the density of high vegetation. Low vegetation structure had a moderate, negative correlation with average canopy height, which is not surprising given that taller shrubs and saplings tend to inhibit dense, low understory growth. In addition, canopy height and density of high vegetation were strongly positively associated. Indeed, capture rates were ∼2× higher in nets associated with a high canopy (>4.5 m) than in those associated with a low canopy (<2.5 m) for Ovenbird, and >6× higher for Wood Thrush. Although the model containing both canopy height and low vegetation structure was never the top model, the two variables explained a substantial amount of variation in capture rates of several groups (HY, AHY postbreeders, Worm-eating Warbler, Red-eyed Vireo, and Wood Thrush). We suggest that tall canopies (shrub-sapling canopy) not only provide additional structural resources, but also protection from aerial predators such as Cooper's Hawks (Accipiter cooperii) and Broad-winged Hawks (Buteo platypterus), common raptors at our sites.
Whereas the abundance of mature-forest HY and AHY postbreeders seemed to be better explained by vegetation structure than by fruit resources, fruit was the most important variable in explaining captures of Scarlet Tanager. Capture rates for Scarlet Tanager were 2.5× greater in nets with high (>600) than in those with low (<100) fruit abundance. This comes as little surprise, because Scarlet Tanagers are known to be highly frugivorous outside the breeding period (Mowbray 1999). In fact, 81% of the Scarlet Tanagers we captured during the postbreeding period in 2003 had fruit stains on their bills. In a pilot study, we found that older clearcuts (10 years postharvest) with a closed canopy and limited fruit were seldom used by Scarlet Tanagers during the postbreeding period, and only two birds were captured in 1,023 net-hours at three sites (A. Vitz and A. Rodewald unpubl. data). Furthermore, as fruits in the clearcuts declined, so did the captures of Scarlet Tanagers. Although the relationship with fruit was strongest for Scarlet Tanagers, we also documented fruit on the bills of Wood Thrush, Ovenbird, and Red-eyed Vireo, which are known to consume fruit outside of the breeding period (Parrish 2000). For Ovenbird, the second- ranked and equally plausible model contained fruit. However, fruit at all sites was dominated by Rubus spp., and birds with extremely small gapes may be less able to capitalize on this temporally abundant but large food resource (Martin 1985). Arthropods also are attracted to fruit, which may further benefit postbreeding birds (Sallabanks and Courtney 1992).
Our findings support the suggestion, frequently made, that postbreeding use of early- successional forests by mature-forest breeders is a response to both vegetation structure and abundant fruit resources (Anders et al. 1998, Vega Rivera et al. 1998b). However, the present study is the first to provide empirical support for vegetation structure as the primary resource for most species. The importance of structural resources seems reasonable, given that birds are extremely vulnerable to predators during the postbreeding period, because juveniles are inexperienced and adults have limited flight capabilities while they molt their flight feathers (Vega Rivera et al. 1998a). Several studies have found that juvenile birds experience high mortality rates from predation (Sullivan 1989, Anders et al. 1997) and, to a lesser extent, starvation (Sullivan 1989). For example, Anders et al. (1997) reported 58% mortality of radio- tagged HY Wood Thrush (51% from depredation and 7% from other causes) during the first four weeks postfledging. Predation rates are frequently highest during the first week postfledging, when birds were learning to fly, and the third week once they gained independence and foraged conspicuously (Sullivan 1989, Anders et al. 1997, Powell et al. 2000). Such vulnerability to predation may render the breeding habitats of many species unsuitable during the post- breeding period and cause postbreeding birds to select habitats where they can forage and rest in the relative safety of dense cover (Anders et al. 1998). This may explain why juvenile birds sometimes disperse into thicker habitats (i.e., riparian thickets, edges, or early-successional forests) shortly after achieving independence. In fact, sharp declines in mortality have been recorded for HY (Anders et al. 1997) and AHY (Powell et al. 2000) Wood Thrush immediately following a shift into denser habitat.
One potentially important factor not examined here is invertebrate resources. As part of another study (on the same sites), we compared arthropod abundance between (large vs. small clearcuts) and within (distance from the mature-forest edge) regenerating clearcuts but found no relationship between arthropod and bird abundances (Vitz and Rodewald 2006). Furthermore, during the postbreeding period, Bowen (2004) found higher bird abundance in canopy gaps than in mature-forest habitat, despite finding fewer foliage-dwelling arthropods in the gaps. However, the efficacy of arthropod sampling has been questioned, because researchers are unable to sample arthropods as perceived by insectivorous birds. As a result, prey availability as measured by a biologist may be quite different from that experienced by a bird (Cooper and Whitmore 1990, Hutto 1990).
Unfortunately, we were unable to model arthropod data at the net level, because samples were combined on the basis of distance to edge, rather than separated by net. Early-successional forests may have a higher abundance of arthropods than mature forests (Jokimäki et al. 1998, Keller et al. 2003), and lepidopterans, heavily preyed upon by birds, may be especially high in regenerating stands (Keller et al. 2003). In fact, arthropods may prefer to graze on leaves of early-successional species (Edwards-Jones and Brown 1993, Farji-Brener 2001) because of their high nutritional value and low levels of secondary compounds (Coley 1983). Thus, the possibility remains that birds select early-successional habitats at least partly for high arthropod abundance.
Our findings support assertions by others that regenerating clearcuts are heavily used by mature-forest songbirds during the postbreeding period (Pagen et al. 2000, Marshall et al. 2003). In fact, a related study detected some of the greatest diversity (32 species) and abundance of mature-forest birds, and the greatest proportion of HY birds (71% of captures), in clearcut stands (Vitz and Rodewald 2006). Because we captured nearly all mature-forest species known to breed in our study area, we suggest that use of regenerating clearcuts is a widespread and general phenomenon in mature-forest birds. Our recapture data (∼5% of individuals recaptured on successive visits) also suggest that some individuals spent substantial time in the clearcuts rather than simply passing through them (Vitz and Rodewald 2006). In light of this, biologists should recognize that early-successional stands may have legitimate conservation value with regard to mature-forest birds during the postbreeding period. Although our study suggests that reasons for this habitat shift vary by species, vegetation structure and fruit resources both seem to play important roles. In addition to microhabitat characteristics, stand-level variables may also influence habitat quality for birds during the postbreeding period. On these same study sites, substantially higher capture rates of mature-forest juveniles and adult postbreeders were found in small (4–9 ha) than in large (13–18 ha) clearcuts, and in clearcut interiors compared with edge areas (Vitz and Rodewald 2006).
The present study illustrates that animals must be studied across their life cycle to identify key habitats and resources. For example, studies focusing solely on breeding success for mature-forest species would emphasize retention of large forest tracts (Rosenberg et al. 1999) but might overlook the possible importance of early-successional habitats during the postbreeding period—an omission that could have consequences for survival and recruitment. Ultimately, what is needed is a careful assessment of the extent to which use of early-successional forests promotes survival or improves condition of birds during the postbreeding period. Future research should evaluate whether access to early-successional forests decreases mortality during the post- breeding period.
Acknowledgments
We are greatly indebted to several field assistants who collected data for this project, including M. Bakermans, J. Berthiaume, K. Higgins, A. Schwarzer, A. Balogh, A. Boone, and B. Baldesberger, Jr. We thank M. Bakermans, R. Gates, T. Grubb, Jr., and D. Swanson for insightful comments that greatly improved the manuscript. Statistical advice was provided by J. Christensen and B. Bishop. We are grateful to P. Rodewald for allowing us to work under his banding permit and logistical support. We thank the Ohio Division of Wildlife and the U.S. Fish and Wildlife Service through the State Wildlife Grant program for funding research, as well as The Ohio State University School of Natural Resources, for research support. We thank D. Swanson (Ohio Division of Wildlife) and W. Lashbrook (Mead-Westvaco) for their assistance in identifying and locating potential study sites. Permission to use study sites was granted by the Ohio Division of Forestry and Mead-Westvaco.
Literature Cited