-
PDF
- Split View
-
Views
-
Cite
Cite
Pawel Brzȩk, Marek Konarzewski, Effect of Refeeding on Growth, Development, and Behavior of Undernourished Bank Swallow (Riparia Riparia) Nestlings, The Auk, Volume 121, Issue 4, 1 October 2004, Pages 1187–1198, https://doi.org/10.1093/auk/121.4.1187
- Share Icon Share
Abstract
Nestlings of aerial insectivores adjust their energetics, behavior, and development of internal organs in response to food shortages and subsequent improvements in feeding conditions. Here, we present results of laboratory refeeding of Bank Swallow (Riparia riparia) nestlings, which were undernourished between 4 and 7 days of age. During a subsequent three-day-long refeeding, their body mass did not catch up with that of individuals continuously fed ad libitum. However, refed young managed to compensate for delayed growth of pectoral muscles, small intestines, and fully restored fat reserves. Their resting metabolic rates and body temperatures increased to values similar to those of nestlings fed ad libitum. In contrast, digestive activity of their guts (quantified as carrier-mediated intestinal brushborder uptake rate of L-proline) was reduced, as compared with the period of undernutrition. Likewise, high locomotor activity observed during food shortage was significantly reduced upon refeeding. Furthermore, differences in body mass between nest mates, which had earlier modulated both locomotor activity and physiological responses to food shortage, had no effect on mobility and physiology of refed nestlings. Our results indicate that even a short, three-day period of refeeding triggered conspicuous acceleration of growth and development of vital internal organs in previously undernourished nestlings. However, that was not accompanied by significant compensatory whole-body growth (defined as a period of above-normal rapid growth, relative to age).
Resumen
Los pichones de las aves insectívoras aéreas son capaces de ajustar su balance energético, su comportamiento y el desarrollo de los órganos internos para adecuarse a los períodos de escasez de alimentos y a las subsecuentes mejoras en las condiciones alimenticias. Presentamos aquí resultados de laboratorio de alimentación post-ayuno de pichones de la golondrina Riparia riparia, los cuales fueron sub-alimentados entre los 4 y 7 días de edad. Durante un período de alimentación post-ayuno de tres días, la masa corporal de estas aves no igualó a la de aquellos individuos alimentados continuamente ad libitum. Sin embargo, los juveniles con alimentación post-ayuno lograron compensar el retraso en el crecimiento de los músculos pectorales, el intestino delgado y el restablecimiento total de las reservas de grasa. Las tasas metabólicas de reposo y las temperaturas corporales aumentaron a valores similares a los de los pichones alimentados ad libitum. En contraste, la actividad digestiva de sus intestinos (cuantificada como la tasa intestinal de absorción de L-prolina mediada por un transportador) se redujo en comparación con el período de sub-alimentación. Del mismo modo, la alta actividad locomotora observada durante los períodos de escasez de alimento se redujo significativamente con la alimentación post-ayuno. Más aún, las diferencias en la masa corporal entre los compañeros de nido, que anteriormente habían modulado tanto las actividades locomotoras como las respuestas fisiológicas a la escasez de alimento, no tuvieron efecto sobre la movilidad y la fisiología de los pichones con alimentación post-ayuno. Nuestros resultados indican que incluso un período corto de tres días de alimentación post-ayuno desencadena una aceleración marcada en el crecimiento y en el desarrollo de los órganos vitales internos en pichones previamente sub-alimentados. Sin embargo, esto no estuvo acompañado por un crecimiento compensatorio significativo de todo el cuerpo (definido como un período de crecimiento rápido por sobre lo normal, relativo a la edad).
Some growing organisms are able to actively adjust their rates of growth and development to fluctuating environmental conditions (“developmental conversion” sensuSmith-Gill 1983; for a review, see Schlichting and Pigliucci 1998). Among birds, such ability is best exemplified by nestlings of aerial insectivores (such as Meropidae, Apodidae, and Hirundinidae), which are capable of slowing their rate of development during periods of inclement weather and resuming the normal rate when feeding conditions improve (Lack and Lack 1951, Bryant 1975, O’Connor 1978a, Emlen et al. 1991).
In a recent study (Brzȩk and Konarzewski 2001), we analyzed physiological and behavioral responses to limited nutrition in Bank Swallow (Riparia riparia) nestlings that were hand reared under laboratory conditions. Food-restricted nestlings not only had lower body-mass increments than individuals fed ad libitum, but also had decreased metabolic rate and body temperature and relatively smaller pectoral muscles, intestines, and fat stores. On the other hand, they had increased locomotor activity (quantified as frequency of crawling into an artificial nest tunnel) and increased intestinal digestive activity (quantified as carrier-mediated intestinal brush-border uptake rate of L-proline). Thus, food-limited Bank Swallow nestlings attempted both to reduce the rate of energy use and to increase energy intake. Interestingly, responses were modified by intensity of sibling competition, related to differences in body mass of nest mates. In the presence of hungry nest mates of similar body mass, underfed individuals had increased locomotor activity and increased uptake rate of proline.
The notion of adaptive developmental plasticity assumes not only the existence of physiological mechanisms allowing for temporary reduction of the rate of growth and development, but also the ability to compensate for the resulting delay when feeding conditions improve (Schew and Ricklefs 1998). Studies on effects of improved feeding conditions in aerial insectivores have mainly been restricted to observations of changes in length of the fledging period (e.g. Lack and Lack 1951, Emlen et al. 1991). However, physiological responses of previously underfed nestlings to improved feeding are poorly known. Although there is a vast literature on physiology of refeeding in domestic chickens (Gallus domesticus; e.g. Plavnik and Hurwitz 1988; Yu et al. 1990; Fontana et al. 1993; Zubair and Leeson 1994a, b), to our knowledge, only three relevant studies have been done on altricial species: European Starling (Sturnus vulgaris; Schew 1995), Song Thrush (Turdus philomelos; Konarzewski et al. 1996), and House Sparrow (Passer domesticus; Lepczyk et al. 1998).
Here, we report results of experimental refeeding of previously undernourished Bank Swallow nestlings. Our aim was to test whether refed individuals can accelerate their growth rate above the level of normally fed individuals to compensate for earlier delay. Undernourished Bank Swallow nestlings had decreased intestinal mass, but simultaneously had increased rates of intestinal uptake of proline (Brzȩk and Konarzewski 2001). Given that growth rate can potentially be limited by digestive capacity of the gastrointestinal tract (Ricklefs et al. 1998) and that birds show a high level of intestinal phenotypic plasticity (Starck 1996), we hypothesized that fueling of compensatory growth would require an earlier investment in the gastrointestinal tract. We expected, therefore, that such investment would result in an increase of the size of intestines or an increase of carriermediated intestinal uptake rates, or both, over values found in individuals of the same age that were fed ad libitum. Furthermore, we expected that refed nestlings would have body temperatures, metabolic rates, and fat reserves restored to levels found in individuals fed ad libitum. We also expected them to compensate for previously delayed growth of pectoral muscles, given that completion of those muscles is essential for successful fledging in aerial insectivores.
In our earlier study (Brzȩk and Konarzewski 2001), we hypothesized that a unique locomotor activity of undernourished Bank Swallow nestlings increased their competitive abilities, enabling them to secure a favorable position near the nest tunnel entrance to intercept food from incoming parents. If so, such activity should be considerably reduced when feeding restriction ends. For the same reason, we expected that differences in body mass of nest mates, which earlier had significantly affected physiological responses to undernutrition, should have only minor or negligible effects on those responses upon refeeding.
Methods
Animals and experimental protocol
All Bank Swallow nestlings used in the present study were earlier subjected to another experimental trial, as described in Brzȩk and Konarzewski (2001). Briefly, 36 four-day-old nestlings were collected in June 1999 from the breeding colony near Bialystok in northeastern Poland, with permission from the nature conservancy authorities (permit no. 4201/296/99). They were taken to the laboratory and maintained in artificial nest cavities with attached nest tunnels, all made of transparent plastic. Except for the tunnel entrances, the artificial nest burrows were completely blinded with cardboard.
The experimental design of the previous (Brzȩk and Konarzewski 2001) and present study is explained in Figure 1. In the previous experiment, we created six artificial broods, each of them containing six nestlings. Two broods were maintained on a limited amount of food (“food-restricted”; hereafter, “FR”). In each of the other four broods, half the nestlings were fed as FR nestlings, whereas their nest mates were fed ad libitum (AL); those two groups within each brood were referred to as “FR/AL” and “AL,“ respectively, in Brzȩk and Konarzewski (2001). Food intake in both FR and FR/AL groups averaged 40% of food consumption of AL nestlings. The experimental design enabled us to study the effect of presence of bigger and well-fed nest mates on food-restricted Bank Swallow nestlings.
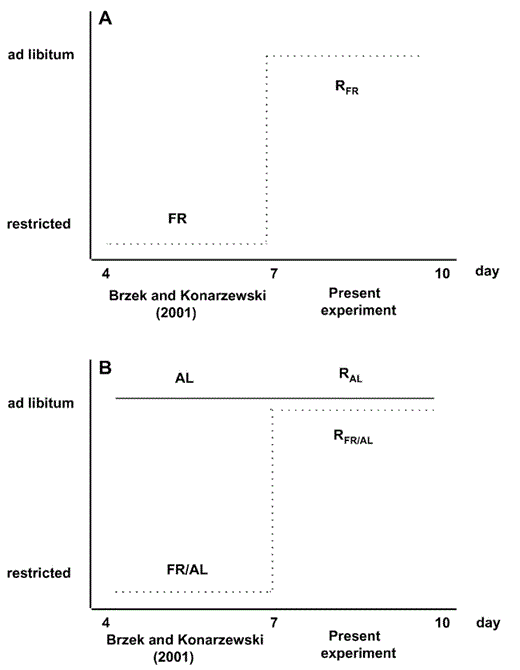
Graphic scheme of feeding regimens applied in the previous (days 4–7) and present experiment (days 7–10). (A) Between 4 and 7 days of age, some nestlings were fed with a limited amount of food (referred to as “FR group” in Brzȩk and Konarzewski 2001). In the present experiment, they were switched to an ad libitum feeding regime applied between 7 and 10 days of age and referred to as “RFR group.” (B) In the previous experiment, half the remaining nestlings (FR/AL group) were fed with a limited amount of food (dashed line). Their nest mates (AL group) were fed ad libitum (solid line). That treatment simulated differences in food supply occurring in asynchronously hatched broods facing food shortage. In the present experiment, nestlings from the former FR/AL and AL group were all fed ad libitum between 7 and 10 days of age, and referred to as “RFR/AL” and “RAL” groups, respectively.
After three days, the previous experiment was terminated, and 14 seven-day-old individuals were subjects of the present study. They were assigned to three broods, one consisting of four nestlings that belonged to the FR group in the previous study (hereafter, “RFR nestlings”); the other two consisting of four and six individuals, respectively. In each of those latter broods, half the nestlings (hereafter, “RFR/AL” nestlings; five individuals) were selected from the previous study's FR/AL group, whereas their nest mates (hereafter, “RAL” nestlings; five individuals) had earlier been fed ad libitum (AL group).
In the present study, nestlings were reared in the same artificial nest cavities as in the earlier experiment. Nest cavities were placed in a climate-controlled chamber at 32°C, 90% relative air humidity, and 16 h light: 8 h dark photoperiod. Ambient temperature was lower than in Brzȩk and Konarzewski (2001), because of better thermoregulatory abilities of older nestlings. Irrespective of earlier treatments, all nestlings subjected to the present experiment were fed according to ad libitum protocol (Fig. 1), as validated and applied in Brzȩk and Konarzewski (2001). Nestlings were removed from artificial nests every 45 min between 0600 and 2200 hours CEST (22 times day−1); then we solicited begging and fed nestlings until they stopped begging. Nestlings were weighed daily to the nearest 0.1 g, to estimate daily body-mass increments. The experiment was terminated upon completion of the third day. We selected a three-day-long refeeding period to restrict the experimental period to the linear phase of growth (Turner and Bryant 1979), when the energy demands of growth per se are highest and, therefore, responses to food-restriction and refeeding are more likely to occur.
During the course of the experiment, one nestling from the RAL group was excluded from the trial because of abnormally low temperature and returned to the natal nest.
Diet composition and food intake
Nestlings were hand fed, alternating between equal amounts of crickets and a special formula designed for nestlings of insectivorous birds (J. Desselberger, pers. comm.). The formula consisted of fresh soft cheese, glucose, rice flour, and maize flour mixed in mass proportion 300: 30:15:12. The mixture was subsequently added to hardboiled chicken eggs (in proportion 3:1) and vitaminenriched with Vitaral (Polfa, Poland). Daily food intake was measured individually for each nestling. To express a nestling's food intake in energy units, we first determined water content of food by drying subsamples at 60°C to constant mass and then determined energy content in a Berthelot-type calorimeter.
Body temperature and locomotor activity
To monitor well-being of nestlings, we measured cloacal temperature (to nearest 0.1°C) of each individual at the artificial nest three times a day, with a 0.5-cm-long thermocouple probe attached to a BAT-12 electronic thermometer (Physitemp Instruments, Clifton, New Jersey). Experimental groups did not differ with respect to body temperature, except during the first 6 h of the experiment. We attributed that difference to the residual effect of the previous experiment, of minor importance for the present study. For the sake of brevity, we report only body temperatures measured after 2.5 days of the experiment, immediately before nestlings were subjected to metabolic trials, completing the experiment.
During the second and third days, we quantified locomotor activity of nestlings as the ratio of the number of feeding bouts when young were found in the nest tunnel to total number of bouts. We excluded data for the first 24 h, to eliminate any possible effect of previous treatment. We pooled together the data on locomotor activity for the second and third day to avoid the possibility of autocorrelations between subsequent days.
Measurements of oxygen consumption
Oxygen consumption was measured upon completion of the third day. We used a TR-1 positive-pressure opencircuit respirometry system (Sable Systems, Las Vegas, Nevada). Individual nestlings were placed in the metabolic chamber (100 mL volume), submerged in a water bath at 33°C, which allowed them to maintain body temperature equal to that measured at the nest (P. Brzȩk pers. obs.). Air-flow rate was set at 200 or 300 mL min−1, depending on mass of the nestling, and controlled with a mass flow meter. The air stream was vented from the metabolic chamber, redried, scrubbed of CO2, and fed at the rate of 50 mL min−1 to the O2 sensor. We estimated resting metabolic rate (RMR) by taking the lowest 2-min value recorded during the second half of a 1-h trial. Metabolic data were analyzed with DATACAN V software (Sable Systems). We calculated oxygen consumption rates using equation (4a) from Hill (1972).
Morphometrics and determination of carcass fat content
Upon completion of metabolic trials, nestlings were sacrificed with ether, and their intestines and pectoral muscles were removed, cleared of adherent fat, and weighed to the nearest 0.001 g. Fat was extracted from the remaining carcasses (minus legs removed for later histochemical analyses) in a Soxhlet extractor, as described in Sawicka-Kapusta (1975).
Intestinal digestive activity
We quantified the digestive activity of nestlings' intestines as brushborder uptake of L-proline, using the everted sleeve technique (Karasov and Diamond 1983). Briefly, small intestines were washed out with cold avian Ringer's solution and cut into 1-cm-long sections. Sleeves were everted, mounted on metal rods, and pre-incubated for 5 min in oxygenated avian Ringer's solution at 37°C. They were subsequently incubated for 2 min at 37°C in Ringer's solution containing 50 mM L-proline (the same nutrient as the [3H] labelled radioactive tracer) and [14C]-polyethylenglycol as an adherent fluid marker. Sleeves were then removed from the rods, put into vials, weighed, solubilized with Soluene-350 (Packard Bioscience, Meriden, Connecticut) mixed with scintillation cocktail (Hionic-Fluor, Packard Bioscience Company), and counted for both radiolabeled compounds. Because of a shortage of radiolabels, we were able to measure uptake rates in the proximal part of intestines only, in which we had earlier found the most distinct responses to differences in food intake and intensity of within-brood competition (Brzȩk and Konarzewski 2001).
We were not able to evaluate histologically the effect of tissue damage incurred by handling on intestinal uptake rates, as recently suggested by Starck et al. (2000). However, all our comparisons involved groups of tissue samples subjected to identical handling. Any bias attributable to tissue damage equally affected all of them; between-group comparisons, therefore, should be reliable. Caution should be exercised, however, if one wishes to use our data for between-species comparisons.
Statistical analyses
Our analyses involved two types of comparisons: (1) between corresponding groups from the previous (Brzȩk and Konarzewski 2001) and present studies (e.g. FR vs. RFR nestlings; because some nestlings used in Brzȩk and Konarzewski [2001] were subjects of the present experiment, we removed them from the Brzȩk and Konarzewski [2001] data set to avoid autocorrelation); and (2) between all three groups in the present experiment.
Many physiological and morphological variables (e.g. body-mass increments, oxygen consumption, and size of internal organs) need to be corrected for body mass prior to comparisons. That is usually done by means of analysis of covariance (ANCOVA), with body mass as a covariate. However, non-overlapping body mass ranges of different groups confound ANCOVA results (for more details, see Brzȩk and Konarzewski 2001). Body mass differed considerably between corresponding groups in the previous (Brzȩk and Konarzewski 2001) and the present experiment. To compare those groups, we therefore computed mass-specific values of traits potentially affected by body mass and analyzed them using nonparametric Mann-Whitney U-tests (Table 1 and Figs. 2–5). We would like to stress that we found no difference, in most cases, between results of parametric ANCOVA and nonparametric tests on mass-specific ratios. In contrast, relatively small between-group differences in body mass of refed nestlings in the present experiment allowed us to analyze their body-mass-affected traits with ANCOVA, followed by post hoc leastsignificant-difference (LSD) tests (Table 2). Also, when the correction for body mass was not necessary, we used either parametric ANOVA (initial and final body mass, body temperature), followed by an LSD test, or a nonparametric Kruskall-Wallis ANOVA, in the case of a skewed data distribution (locomotor activity).
Significance of differences in energy intake, body-mass increment,resting metabolic rate (RMR), body temperature, carcass fat content, pectoral muscle mass, small-intestine mass, intestinal uptake rate of L- proline, and locomotor activity of RFR, RFR/AL, and RAL groups of Bank Swallow nestlings in the present study and in the corresponding groups of nestlings from Brzȩk and Konarzewski (2001). Differences were tested by Mann-Whitney U-test. Superscripts indicate critical levels of significance calculated with false-discovery-rate procedure (FDR; k = 9): 1 = 0.05, 2 = 0.044,3 = 0.039,4 = 0.033, 5 = 0.028,6 = 0.022, 7 = 0.017, 8 = 0.011, 9 = 0.006. Asterisks indicate significant differences.
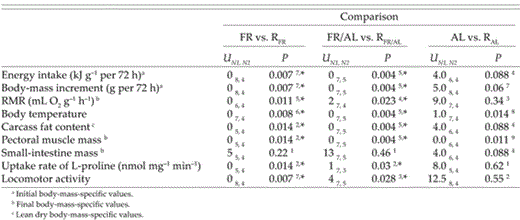
Significance of differences in energy intake, body-mass increment,resting metabolic rate (RMR), body temperature, carcass fat content, pectoral muscle mass, small-intestine mass, intestinal uptake rate of L- proline, and locomotor activity of RFR, RFR/AL, and RAL groups of Bank Swallow nestlings in the present study and in the corresponding groups of nestlings from Brzȩk and Konarzewski (2001). Differences were tested by Mann-Whitney U-test. Superscripts indicate critical levels of significance calculated with false-discovery-rate procedure (FDR; k = 9): 1 = 0.05, 2 = 0.044,3 = 0.039,4 = 0.033, 5 = 0.028,6 = 0.022, 7 = 0.017, 8 = 0.011, 9 = 0.006. Asterisks indicate significant differences.
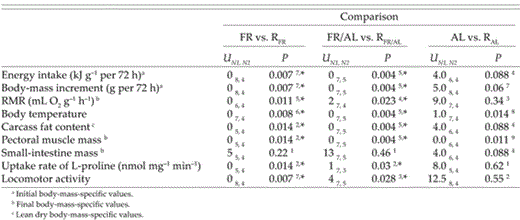
Energy intake, initial body mass, body-mass increment, final body mass, resting metabolic rate (RMR), body temperature, carcass fat content, pectoral muscle mass, mass of small intestines, intestinal uptake rate of L-proline, and locomotor activity of nestlings from the three experimental groups. Means (or least-square means from ANCOVA) ±SE were compared with ANOVA (*), ANCOVA (§), or Kruskall-Wallis test (‡). For all tests, P values and critical levels of significance α are calculated by means of the false-discovery-rate procedure (FDR; k = 11). Means marked with superscript letters (a, b) differ significantly according to least- significant-difference (LSD) test.

Energy intake, initial body mass, body-mass increment, final body mass, resting metabolic rate (RMR), body temperature, carcass fat content, pectoral muscle mass, mass of small intestines, intestinal uptake rate of L-proline, and locomotor activity of nestlings from the three experimental groups. Means (or least-square means from ANCOVA) ±SE were compared with ANOVA (*), ANCOVA (§), or Kruskall-Wallis test (‡). For all tests, P values and critical levels of significance α are calculated by means of the false-discovery-rate procedure (FDR; k = 11). Means marked with superscript letters (a, b) differ significantly according to least- significant-difference (LSD) test.

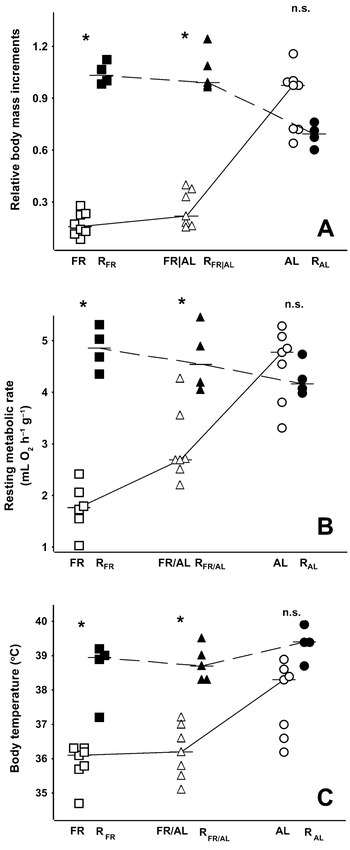
(A) Body-mass increments (expressed as the ratio of absolute body-mass increment to initial body mass at the beginning of the experiment), (B) final mass-specific resting metabolic rate (RMR), and (C) body temperature in Bank Swallow nestlings reported by Brzȩk and Konarzewski (2001 and present study). Horizontal bold segments denote median values, connected with dashed lines (present study) and solid lines (Brzȩk and Konarzewski 2001) to indicate trends. Open symbols denote individual nestlings from Brzȩk and Konarzewski (2001): square = FR, triangle = FR/AL, circle = AL nestlings. Closed symbols indicate nestlings from present experiment: square = RFR, triangle = RFR/AL, circle = RAL nestlings. Stars indicate significant difference between corresponding groups from Brzȩk and Konarzewski (2001) and present study.; n.s. = difference not significant by Kruskall-Wallis test followed by false-discovery-rate procedure.
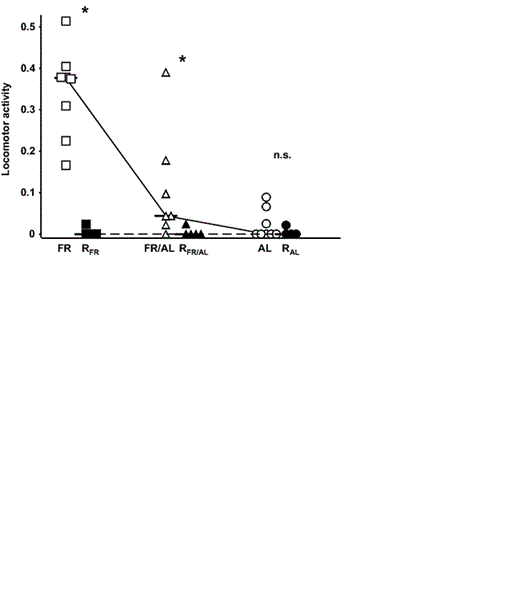
Locomotor activity (quantified as the ratio of number of feeding bouts when young were found in the nest tunnel to total number of bouts) of Bank Swallow nestlings reported by Brzȩk and Konarzewski (2001) and present study. For explanation of symbols used, see Figure 2 caption.
We used the conventional level of significance, α = 0.05. Our statistical analyses involved several multiple comparisons. Therefore, we adjusted the conventional level of significance by applying the false-discovery-rate procedure (see Curran-Everett 2000). That newly developed procedure ensures that the expected ratio of null hypotheses rejected mistakenly to the total number of hypotheses rejected is not >0.05. Briefly, for each set of multiple comparisons (e.g. FR vs. RFR nestlings; see Table 1), we ranked the results of k tests by decreasing magnitude of obtained P levels. We then calculated the critical significance level αi (where i = k, k − 1, …, 1) for each comparison as αi = (i/k) × 0.05. We report as significant all comparisons for which Pi ≤ αi (see Curran-Everett 2000 for more details) and provide all critical significance levels in Tables 1 and 2. Tests were carried out using STATISTICA software (StatSoft, Tulsa, Oklahoma).
Results
Energy intake and growth
All three groups of nestlings in the present study had significantly higher energy-intake than the corresponding groups in Brzȩk and Konarzewski (2001) (ANOVA followed by Tukey test, P < 0.001, in all comparisons). However, when expressed as food intake:body mass ratio, those differences were significant for both RFR and RFR/AL groups, but not for RAL nestlings (Table 1). The nestlings of three groups in the present experiment had significantly different energy intakes during the three-day refeeding period (ANOVA, F = 7.77, df = 2 and 10, P = 0.009; initial body mass was not significant as a covariate; Table 2). Energy intake of both refed groups did not differ (LSD test, P = 0.1) and was significantly lower than that of the RAL group (RFR vs. RAL group: P = 0.003; RFR/AL vs. RAL group: P = 0.04).
At the beginning of the present study, body masses of nestlings in the three groups were significantly different (ANOVA, F = 15.33, df = 2 and 11, P = 0.0007). They did not differ between RFR and RFR/AL groups, but were lower than in RAL nestlings (Table 2). Relative-body-mass increments of RFR and RFR/AL nestlings were higher than those of the corresponding groups of the previous experiment (Table 1 and Fig. 2A). However, there was no difference between RAL and AL individuals.
When analyzed with ANCOVA, body-mass increments did not differ between groups of nestlings in the present study (F = 2.11, df = 2 and 9, P = 0.18; initial body mass was significant as a covariate; Table 2). As a result, the initial pattern of between-group differences in body mass still held after three days of refeeding (ANOVA, F = 12.27, df = 2 and 10, P = 0.002; Table 2), with no difference in final body mass between nestlings of both refed groups (P = 0.87), which were still lighter than RAL individuals (in both cases, P < 0.005). However, mass-specific increments (i.e. expressed as ratio of body-mass increment to initial body mass) of RAL nestlings tended to be lower than those of refed groups (Kruskall-Wallis test, H = 7.83, P = 0.02; Fig. 2A), though the difference did not reach statistical significance when we applied the false-discovery-rate procedure to all data presented in Table 2 (the critical level of significance is then 0.018).
Resting metabolic rate and body temperature
Nestlings in the RFR and RFR/AL groups had significantly higher RMR than the FR and FR/AL individuals in Brzȩk and Konarzewski (2001), whereas the differences between RMR of RAL and AL nestlings did not reach statistical significance (Table 1 and Fig. 2B). The RFR and RFR/AL groups had significantly higher body temperature than the corresponding groups in Brzȩk and Konarzewski (2001), whereas that difference did not reach significance for the RAL group (Table 1 and Fig 2C). There were no differences between either RMR (ANCOVA, F = 2.12, df = 2 and 8, P = 0.18; final body mass was significant as a covariate) or body temperature (ANOVA, F = 1.53, df = 2 and 10, P = 0.26) of the RFR, RFR/AL, and RAL nestlings (Table 2).
Fat content and mass of internal organs
Comparisons of FR with RFR nestlings and of FR/AL with RFR/AL nestlings revealed that refeeding resulted in significant accumulation of fat stores (Table 1 and Fig. 3A). In contrast, there was no statistical difference in fat accumulation between RAL and AL individuals (Table 1 and Fig. 3A). The RFR and RFR/AL groups had significantly larger pectoral muscles than the corresponding groups in Brzȩk and Konarzewski (2001), whereas that difference did not reach significance for the RAL group (Table 1 and Fig. 3B). On the other hand, there was no betweenstudy difference in mass of small intestines (Table 1 and Fig. 3C).
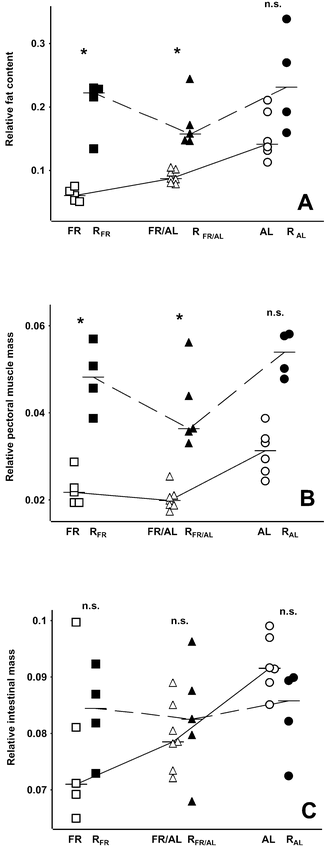
(A) Lean dry body-mass-specific fat mass, (B) body-mass-specific pectoral muscle mass, and (C) body-mass-specific intestinal mass in Bank Swallow nestlings reported by Brzȩk and Konarzewski (2001) and present study. For explanation of symbols used, see Figure 2 caption.
There was no difference in mass of fat among experimental groups in the present study (ANCOVA, F = 2.02, df = 2 and 9, P = 0.19; lean dry body mass was significant as a covariate; Table 2). Likewise, when differences in body mass were accounted for, nestlings did not differ with respect to pectoral muscle mass and intestinal mass (ANCOVA, F = 3.57, df = 2 and 9, P = 0.07 and F = 0.1, df = 2 and 9, P = 0.91, respectively; final body mass was significant as a covariate; Table 2).
Intestinal brush-border uptake of L-proline
Intestinal brush-border uptake of L-proline of RFR and RFR/AL nestlings was significantly lower than that of FR and FR/AL young (Table 1 and Fig. 4). In contrast, there was no difference between uptake rates of RAL and AL groups. Likewise, there was no difference between uptake rates of the three experimental groups in the present study (ANOVA, F = 1.21, df = 2 and 8, P = 0.35; Table 2).
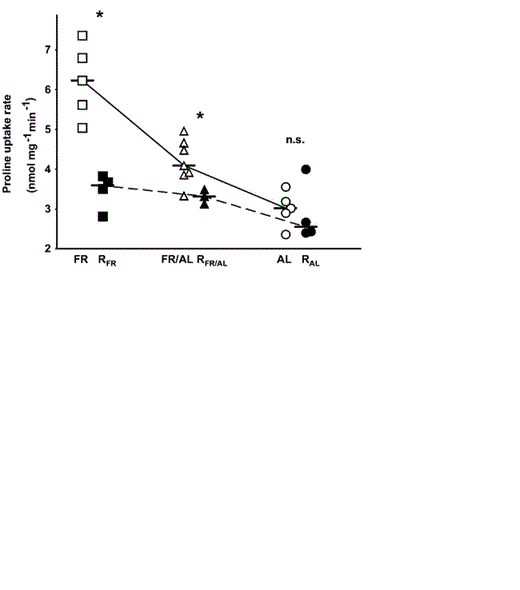
Intestinal L-proline uptake rate in proximal part of small intestines of Bank Swallow nestlings reported by Brzȩk and Konarzewski (2001) and present study. For explanation of symbols used, see Figure 2 caption.
Locomotor activity
Refeeding resulted in conspicuous reduction of locomotor activity in RFR and RFR/AL nestlings, as compared with the corresponding groups of food-restricted nestlings in the previous study (Table 1 and Fig. 5). In contrast, locomotor activity of RAL nestlings remained at a low level, comparable to that observed earlier in the AL group. There was no difference in locomotor activity between the three experimental groups in the present experiment (Kruskall-Wallis test, H = 0.015, P = 0.99; Table 2).
Discussion
Food intake and growth
Despite ad libitum feeding, energy intake of both RFR and RFR/AL nestlings was lower than that of RAL individuals permanently fed ad libitum. That contrasts with increased food intake of hand fed re-alimented American Kestrels (Falco sparverius) (Negro et al. 1994) and House Sparrows (Lepczyk and Karasov 2000). It is unlikely, however, that this discrepancy results from inadequate hand feeding in our study, because by using the same feeding technique we were able to sustain high food intake in RAL nestlings. Furthermore, high locomotor activity, observed in undernourished individuals (Brzȩk and Konarzewski 2001), was almost totally suppressed by refeeding (Fig. 5). Most importantly, however, refeeding of RFR and RFR/AL nestlings resulted in rapid accumulation of substantial fat stores and fast growth of pectoral muscles, which would be unlikely under inadequate feeding.
Relatively low food-intake by refed nestlings precluded them from “catching up” with the body mass of the RAL group during the three-day refeeding. However, Bank Swallows are characterized by a 22-day nestling period, with peak body mass already attained at 15 days of age (Turner and Bryant 1979). It is therefore possible that under longer periods of favorable feeding conditions, retarded individuals can attain normal body mass, as suggested by the trend apparent in Fig. 2A. The ability of nestlings of another aerial insectivore, the Tree Swallow (Tachycineta bicolor), to regain normal body mass in the course of a seven-day refeeding (Wiggins 1990) also supports that possibility.
Body temperature and resting metabolic rate
We hypothesized that frequent and unpredictable fluctuations in food abundance selected for mechanisms enhancing temporary energy savings in Bank Swallow nestlings. Indeed, nestlings responded to food restriction with a reduction in body temperature and RMR (Brzȩk and Konarzewski 2001). In further agreement with the above hypothesis, refeeding resulted in significant increases in nestlings' metabolic rate and body temperature, which finally matched values observed in RAL nestlings. Those results agree with adjustments of body temperature and metabolic rate to food availability observed in nestlings of other aerial insectivores: Common Swift (Apus apus; Koskimies 1948, in Prinzinger and Siedle 1988) and House Martin (Delichon urbica; Prinzinger and Siedle 1988). However, that is in a stark contrast with the responses of typical altricial nestlings, such as European Starlings (Schew 1995) and Song Thrushes (Konarzewski and Starck 2000), which attempted to maintain normal body temperature and metabolic rate during undernutrition. We suggest that this difference reflects unpredictability of the food base for aerial insectivores, which selects for high flexibility of energy budgets in their young (Schew and Ricklefs 1998).
Intestinal mass and function
Food restriction resulted in a significant decrease of relative mass of intestines in Bank Swallow nestlings (Brzȩk and Konarzewski 2001). After three days of refeeding, we found no further differences between groups of previously food-restricted and ad libitum-fed young (Table 2), which suggests that refeeding triggered some increase of intestinal mass. That increase, however, was very moderate, given that it did not result in significant differences between relative intestinal mass of the corresponding groups of nestlings from the present and previous studies (Fig. 3C). Because most of the nutrients are absorbed passively in the avian intestine (Karasov et al. 1996, Afik et al. 1997), a conspicuous increase in digestive capacity is likely to be associated with increased luminal surface area of the intestines and, therefore, conspicuous increase in intestinal mass. We hypothesize that conspicuous acceleration of growth rate would require an increase of digestive capacities over the values observed in RAL nestlings (see also Palo et al. 1995). Thus, a limited increase in intestinal mass of refed nestlings is consistent with the lack of compensatory whole-body growth.
In contrast to the effect on intestinal mass, food restriction increased digestive activity quantified as intestinal uptake rates of proline (Brzȩk and Konarzewski 2001). In the present study, we found that previously undernourished nestlings responded to refeeding with significant reduction of intestinal uptake rates to levels typical of individuals continuously fed ad libitum (Fig. 4). Thus, undernourished Bank Swallows were able to respond to improved feeding conditions by bringing digestive activity into line with values characteristic of normally growing individuals (Table 2). In our view, that is yet another indication of their developmental plasticity. We hypothesize that the carrier-mediated transport may become particularly important under food limitation, when active scavenging of the sparse nutrients from the luminal volume may increase the chances of survival. On the other hand, the active transport may be safely reduced under ad libitum conditions, when the passive paracellular pathway suffices to cover the majority of nutritional needs (Karasov et al. 1996, Afik et al. 1997).
Fat mass and pectoral mass
During the three-day refeeding period, young Bank Swallows were able to fully restore fat deposits earlier depleted by food restriction (Table 2). We hypothesize that rapid restoration of fat reserves can be adaptive in the nestlings of aerial insectivores, such as Bank Swallows, and may be crucial for survival through possible subsequent periods of food shortage caused by inclement weather (e.g. O’Connor 1977, 1978b; Bryant and Gardiner 1979).
Refeeding also enabled undernourished nestlings to compensate for delayed growth of pectoral muscles (Fig. 3B). In contrast, food restriction and refeeding had little effect on growth of pectoral muscles in Song Thrush nestlings (Konarzewski et al. 1996). Such different responses suggest that, unlike species exploiting predictable food resources, aerial insectivores can adjust growth of pectoral muscles to fluctuating feeding conditions. We hypothesize that the great flexibility of their growth may be essential for successful fledging and presumably parallels development of other organs related to flight ability (e.g. primary feathers), which is also adjusted to food availability (Lack and Lack 1951, McCarty and Winkler 1999).
Locomotor activity and sibling competition
In our earlier study (Brzȩk and Konarzewski 2001), we suggested that high locomotor activity in Bank Swallow nestlings (quantified as frequency of crawling into artificial nest tunnel) was triggered by restricted food consumption. That is corroborated by the results of the present study, because refeeding significantly suppressed locomotor activity of previously undernourished individuals (Table 1 and Fig. 5). Furthermore, in our earlier study, we demonstrated that size hierarchy of hungry nestlings not only affected their locomotor activity, but also modulated their physiological responses to food shortage, such as fat accumulation, body temperature, and digestive activity of the gut (Brzȩk and Konarzewski 2001). However, RFR and RFR/AL nestlings did not differ with respect to physiological responses to refeeding (Table 2), even though the latter were reared with much larger RAL siblings. All of which suggests that between-sibling interactions significantly affect both locomotor activity and physiology of nestlings when resources are limited, and become negligible when feeding conditions improve (see also Cook et al. 2000).
Controversy of compensatory growth
The nature of avian compensatory growth (i.e. accelerated growth relative to age or physiological state, usually associated with refeeding after a period of malnutrition; Schew and Ricklefs 1998) is still controversial. It was reported in domestic chickens (e.g. Plavnik and Hurwitz 1985; Zubair and Leeson 1994a, b; but see Rosebrough and McMurtry 1993, Zubair and Leeson 1996) and in young American Kestrels (Negro et al. 1994). However, there was no evidence for compensatory growth in nestlings of typical altricial passerines: European Starling (Schew 1995), Song Thrush (Konarzewski et al. 1996), and House Sparrow (Lepczyk and Karasov 2000). Likewise, we did not observe the phenomenon in Bank Swallows, in that body-mass increments of refed nestlings did not significantly exceed those of nestlings continuously fed ad libitum (Table 2).
It is important to note, however, that three-day refeeding of retarded nestlings was clearly sufficient for them to catch up in relative size of several body parts (pectoral muscle, intestines, fat stores), as well as in RMR and body temperature of the RAL group (Table 1). Thus, although whole-body growth of refed Bank Swallow nestlings may not meet the criterion of compensatory growth, the rates of change in body proportions and metabolic processes may. We therefore propose to distinguish between “compensatory growth” and “compensatory development.” Whereas the first term applies to body-mass increments, the second term refers to faster development of some body parts and physiological processes than in normally fed individuals, which in our study is best exemplified by accelerated growth of pectoral muscles (Table 1 and Fig. 3B).
It is also important to note that refed nestlings were not only able to increase the mass of pectoral muscles at a rate exceeding that observed in individuals fed ad libitum, but at the same time increased RMR and body temperature. That seems incompatible with the notion of a general trade-off between functional maturity of a muscle (e.g. shivering thermogenesis) and its ability to grow (Ricklefs and Webb 1985; Ricklefs et al. 1994, 1998). Furthermore, it suggests that even when feeding conditions are extremely favorable, as in the case of RAL nestlings fed ad libitum, muscles still do not use their full growth potential. However, the typical pattern of development of pectoral muscles in Bank Swallows consists of slow growth during the first week of life, followed by rapid mass increase and changes in enzymatic activity when body mass exceeds 12 g (Marsh and Wickler 1982). Thus, we cannot exclude the possibility that pectoral muscles of Bank Swallows initially grow at a submaximal rate, allowing for short-term adjustment to feeding conditions. Timing of fledging can ultimately be constrained by some other factor (e.g. feather growth; see Johnston 1993).
We conclude that undernourished nestlings of aerial insectivores are capable of accelerating growth and development of vital internal organs when feeding conditions improve. However, at least during the three-day period of refeeding applied in the present experiment, they were unable to demonstrate significant compensatory whole-body growth, defined as a period of above-normal rapid growth, relative to age.
Acknowledgments
We thank E. Burak, A. Gȩbczyński, M. Lewoc, A. Roszkowska, U. Szymska, and J. Taylor for skillful help in laboratory trials. We thank two anonymous reviewers for their thoughtful comments. The study was supported by Polish State Committee for Scientific Research (KBN) grant 6 PO4F 054 14 to P.B. and KBN grant 6 P204 072 to M.K.
Literature Cited