-
PDF
- Split View
-
Views
-
Cite
Cite
Ruth B. Smith, Ellis C. Greiner, Blair O. Wolf, Migratory Movements of Sharp-Shinned Hawks (Accipiter Striatus) Captured in New Mexico in Relation to Prevalence, Intensity, and Biogeography of Avian Hematozoa, The Auk, Volume 121, Issue 3, 1 July 2004, Pages 837–846, https://doi.org/10.1093/auk/121.3.837
- Share Icon Share
Abstract
Previous interspecific studies have shown that migratory birds have a higher prevalence of blood parasites than nonmigratory birds. It is thought that birds that move greater distances are exposed to a larger parasite fauna, because of uneven distribution of vectors that carry such parasites. Current hypotheses also suggest that the stress of migration may allow parasites to persist, by weakening the immune system and allowing latent infections to recur. We captured migrating hatching-year Sharp-shinned Hawks (Accipiter striatus) in New Mexico and estimated natal latitudes of each individual by analyzing stable-hydrogen isotope ratios of feathers. That enabled us to estimate distances traveled up to point of capture. We also collected blood to quantify hematozoan infection prevalence and intensity. We related distance traveled to prevalence and intensity of hematozoan infections. Contrary to previous hypotheses, our results show no relationship between distance traveled by migrants and prevalence and intensity of hematozoan infections. Of 75 individuals examined, 24% were infected with Leucocytozoon toddi, 37% with Haemoproteus elani, and 5% with H. janovyi. To our knowledge, this is the first documented occurrence of H. janovyi in North America and in Sharp-shinned Hawks. Our stable-hydrogen isotope analyses indicated that H. janovyi was detected only in birds that originated in southwestern North America, which may be of significance in regard to biogeography of that parasite species.
RESUMEN
Estudios previos a nivel interespecifico han mostrado que las aves migratorias tienen una mayor prevalencia de parásitos sanguíneos que las aves no migratorias. Se piensa que debido a la distribución desigual de los vectores que transportan los parásitos, las aves que se mueven mayores distancias están expuestas a una mayor fauna de parásitos. Las hipótesis actuales también sugieren que el estrés producido por la migración puede permitir la persistencia de los parásitos, debido a la debilitación del sistema inmunológico y permitiendo que infecciones latentes vuelvan a ocurrir. En Nuevo México capturamos halcones de la especie Accipiter striatus que eclosionaron en el año y que se encontraban migrando. Estimamos la latitud natal de cada individuo analizando el cociente de isótopos estables de hidrógeno en las plumas, lo que nos permitió estimar las distancias viajadas hasta el punto de captura. También colectamos sangre para cuantificar la prevalencia e intensidad de la infección por hematozoos. Relacionamos la distancia viajada con la prevalencia e intensidad de la infección por hematozoos. De manera contraria a las hipótesis previas, nuestros resultados no mostraron relacón entre la distancia viajada por los migrantes y la prevalencia e intensidad de las infecciones por hematozoos. De un total de 75 individuos examinados, el 24% estuvo infectado por Leucocytozoon toddi, el 37% por Haemoproteus elani y el 5% por H. janovyi. Según nuestro conocimiento esta es la primera documentación de la presencia de H. janovyi en Norte América y en el halcón A. striatus. Nuestros análisis de isótopes estables de hidrógeno indicaron que H. janovyi fue detectado sólo en aves originarias del suroeste de Norte América, lo que puede ser significativo con relación a la biogeografía de esta especie parásita.
Several studies have shown that avian blood parasites have negative effects on fitness and survival of their hosts. For example, Sanz et al. (2001) found that Pied Flycatchers (Ficedula hypoleuca) infected with blood parasites had (1) a higher probability of deserting eggs, (2) reduced hatching success, and (3) difficulty thermoregulating during incubation, compared with non-infected Pied Flycatchers. Other studies have shown that birds infected with blood parasites produce smaller clutches (Korpimäki et al. 1995) and fewer fledglings (Møller et al. 1990; but see Sanz et al. 2001). Hematozoa have also been found to compromise the immune system (Ots and Hörak 1998) and cause anemia in birds (Atkinson and van Riper 1991). Furthermore, hematozoan infections of high intensity have been associated with death in some species (Herman et al. 1975, Julian and Galt 1980). Given the effects that blood parasites can have on their hosts, it is important to consider host-parasite interactions when assessing birds’ life-history strategies.
Abundances of vectors responsible for infecting birds can vary across time and space (Greiner et al. 1975, Merilä et al. 1995) and may result in temporal and spatial variations in prevalence and intensity of hematozoan infections in local populations (Greiner et al. 1975, Merilä et al. 1995, Sol et al. 2000, Deviche et al. 2001, Schrader et al. 2003). Interspecific studies suggest that migratory birds tend to have higher prevalence of hematozoan infections than nonmigratory birds (Bennett and Fallis 1960, Figuerola and Green 2000). That may be attributable to prolonged exposure to blood parasites, or introduction to a larger parasite fauna, or both. Møller and Erritzoe (1998) found that migratory birds had larger spleens and bursa of Fabricius than nonmigratory birds—a response, they suggested, to being exposed to a larger parasite fauna. Other researchers (Valkiunas 1993, Møller and Erritzoe 1998) have suggested that the stress of migration may allow latent infections to resurface.
We examined the prevalence and intensity of hematozoan infections in hatching-year (HY) Sharp-shinned Hawks (Accipiter striatus) migrating through western North America during fall 2001, relative to their natal latitudes and distance traveled during migration. We hypothesized that hawks flying longer distances during migration would have a higher prevalence and intensity of hematozoan infection. We also identified the hematozoan species infecting the migrants, to better understand the biogeography of those parasite species and the origins of infection.
Methods
Trapping and banding
During fall 2001, migrating HY Sharp-shinned Hawks were trapped and banded at a hawk migration research site operated by HawkWatch International. The research site is located in the Manzano Mountains of the Cibola National Forest in New Mexico (34.71°N, 106.41°W; see DeLong and Hoffman 1999, for more details on site description). All research was conducted with approval of the University of New Mexico Institutional Animal Care and Use Committee. Three types of net (mist-, bow-, and dhogaza nets) were baited with live lures (Rock Doves [Columba livia], Ringed Turtle-Doves [Streptopelia risoria], and House Sparrows [Passer domesticus]) to trap migrants. Once captured, all hawks were fitted with a U.S. Fish and Wildlife band, and morphological measurements were taken. We drew blood from the brachial vein and used a single drop of whole blood to make a smear on a microslide; 1–3 smears were made from each individual hawk. In addition, a small clipping (approximately 1–2 cm) was taken from a secondary flight feather for stable-hydrogen isotope analysis.
Stable-hydrogen isotope analysis
Recent studies have shown a relationship between stable-hydrogen isotope ratios in bird feathers and local precipitation where the feathers were grown (Chamberlain et al. 1997, Hobson and Wassenaar 1997, Meehan et al. 2001, Kelly et al. 2002, Rubenstein et al. 2002, Lott et al. 2003). That phenomenon results from spatial patterns in stable-hydrogen isotope ratios (δD) of rainwater in North America, whereby δD values of rainwater decrease with increasing latitude (Araguas et al. 2000). Rainwater δD values undergo an initial fractionation when incorporated into plant tissue (White 1988) and remain fairly unchanged when incorporated into the rest of the food chain, including the keratin of growing feathers from primary and secondary consumers (Chamberlain et al. 1997, Hobson and Wassenaar 1997, Meehan et al. 2001, Lott et al. 2003). Therefore, feather δD values of immature Sharp-shinned Hawks are biological markers that indicate the birds’ natal latitudes.
We used standard methods described in Smith et al. (2003) to calculate feather δD values from migrants. We calibrated our results with keratin standards that were analyzed using methods from Wassenaar and Hobson (2003). Here, therefore, feather δD values represent non-exchangeable hydrogen. All stable-hydrogen isotope analyses were conducted at the University of New Mexico Stable Isotope Laboratory. The δD values are reported in parts per thousand (‰). Precision of analyses was δD ± 5‰, based on repeated measurements of internal laboratory standards.
Blood parasites
Blood smears were allowed to airdry, then fixed in 100% methanol and stored at room temperature until needed for lab analyses. In the lab, all smears were stained using a Giemsa stain. To determine prevalence, we chose one slide from each hawk sample, basing our selection on quality of stain and smear. Smears were examined for 25 min at 100×, using oil immersion for the presence or absence of Haemoproteus and Leucocytozoon species. Because thickness of smears varied along the length of slides, only portions of each slide were examined for presence of hematozoa. Hematozoa can be difficult to detect in some individuals, using blood-smear and microscopy techniques (Jarvi et al. 2001); thus, we note that individuals referred to as “uninfected” are those in which hematozoa were not detected.
In all slides where parasites were found, we measured intensity. To determine intensity of infection for each hawk, 2,000 erythrocytes were counted in 40 replicates of 50 erythrocytes at 100×. That was done for each hematazoan genus found in an individual host. Therefore, “intensity” is defined as number of erythrocytes infected by a hematozoan genus per 2,000 erythrocytes counted for each individual. If no infected cells were counted in assessing intensity, that sample was assigned “0.5 per 2,000” so that it could be included in statistical analyses. Because infected erythrocytes can be unevenly distributed along the length of the blood smear, a die was rolled to determine number of fields skipped between each field examined. That ensured a nonbiased sampling of the blood-smear area on each microslide examined. If a field of view was inadequate for assessing intensity, we skipped that field and measured the next adequate field. Identification of parasite species were made using Peirce et al. (1990).
Statistical analyses
Logistic regression was used to determine the relationship between feather δD values (estimated natal latitudes) and prevalence of hematozoa detected. Linear regression was used to determine the relationship between feather δD values and intensity of infections for birds with hematozoa detected. Logistic and linear regression were performed using S-PLUS (Insightful, Seattle, Washington). Ordinal logistic regression was used to relate feather δD values to total number of hematozoan species detected. Ordinal logistic regression was performed using SAS-JMP (SAS Institute, Cary, North Carolina).
Results
Blood parasites
Blood smears from the same 75 Sharp-shinned Hawks were examined for blood parasites; 31 of 75 individuals (41%) were infected with hematozoa. Haemoproteus species were detected in 28 of the 75 hawks (37%; Table 1); all 28 were infected with H. elani, and four were also infected with H. janovyi. Intensity of Haemoproteus infection ranged from 1 per 2,000 erythrocytes to 132 per 2,000 (median = 13 per 2,000). Eighteen of 75 individuals (24%) were infected with L. toddi (Table 1). Intensity of L. toddi ranged from 0.5 per 2,000 to 4 per 2,000 (median = 0.5 per 2,000. Three individuals were infected with all three species, H. elani, H. janovyi, and L. toddi.
Number of Haemoproteus and Leucocytozoon infections detected in hatching-year Sharp-shinned Hawks migrating through North America during fall 2001. Percentages represent prevalence of infections (prevalence = number of infected individuals divided by total number of individuals examined).

Number of Haemoproteus and Leucocytozoon infections detected in hatching-year Sharp-shinned Hawks migrating through North America during fall 2001. Percentages represent prevalence of infections (prevalence = number of infected individuals divided by total number of individuals examined).

Stable-isotope and hematozoan analyses
Feathers from 75 migrating HY Sharp-shinned Hawks were analyzed for their δD values. Those values ranged from −45‰ to −173‰ VSMOW (Vienna Standard Mean Ocean Water). More-negative feather δD values indicate natal origins of relatively higher latitudes and relatively longer migration-distance traveled up to point of capture; higher feather δD values indicate natal origins of relatively lower latitudes and shorter distance traveled.

Those precipitation values were then used in a GIS-based (geographic information system) model (Meehan et al. 2004; see Acknowledgments) to generate a map depicting potential natal ranges of infected Sharp-shinned Hawks (Fig. 1).

Map of potential natal ranges of migrating hatching-year Sharp-shinned Hawks trapped in New Mexico in fall 2001. Dark gray areas show estimated natal ranges for individuals infected with H. elani. Light gray areas illustrate natal ranges of individuals infected with H. elani and L. toddi. Black areas indicate natal ranges of those infected with H. elani, L. toddi, and H. janovyi. Dashed line represents the Rocky Mountain flyway, used by hawks migrating through central New Mexico in fall (Hoffman et al. 2002). Estimates of natal ranges were derived from stable-hydrogen isotope analyses of feathers (see text for details on those analyses and methods used for generating map).
No relationship was found between presence of L. toddi infections and feather δD values (χ2 = 1.262, df = 1, P = 0.26), sex (χ2 = 0.118, df = 1, P = 0.73), or interaction between feather δD values and sex (χ2 = 0.961, df = 1, P = 0.33; Fig. 2A). In addition, there was no relationship between presence of H. elani infections and feather δD values (χ2 = 0.133, df = 1, P = 0.72), sex (χ2 = 0.548, df = 1, P = 0.46), or interaction between feather δD values and sex (χ2 = 0.015, df = 1, P = 0.90; Fig. 2B). Finally, no relationship was apparent between presence of H. janovyi infections and feather δD values (χ2 = 1.548, df = 1, P = 0.21), sex (χ2 = 1.296, df = 1, P = 0.26), or interaction between feather δD values and sex (χ2 = 0.012, df = 1, P = 0.91; Fig. 2C). When all three hematozoan species were combined, there was no relationship between total number of hematozoan species detected and feather δD values (χ2 = 1.57, df = 1, P = 0.21), sex (χ2 = 0.442, df = 1, P = 0.51), or interaction between feather δD values and sex (χ2 = 0.389, df = 1, P = 0.53; Fig. 2D).
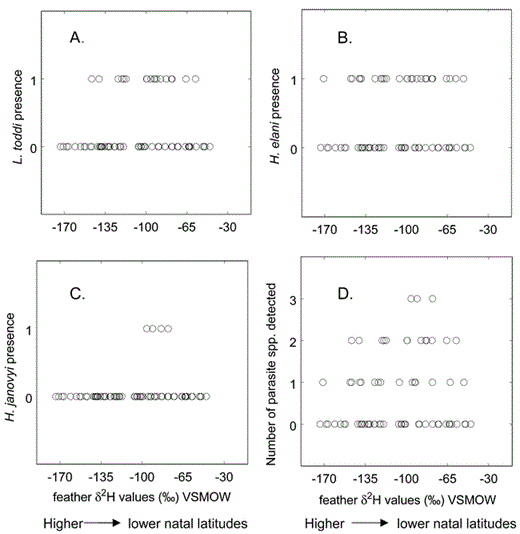
Relationship between feather δD values and prevalence of (A) L. toddi, (B) H. elani, and (C) H. janovyi infections in migrating hatching-year Sharp-shinned Hawks (0 = no detection of parasites; 1 = parasite detected). (D) Relationship between feather δD values and total number of hematozoa species detected. Feather δD values were generated by stable-hydrogen isotope analyses (see text) and are reported in parts per thousand (‰), VSMOW.
We also found no relationship between intensity of L. toddi infections and feather δD values (F = 1.652, df = 1 and 14, P = 0.22), sex (F = 1.057, df = 1 and 14, P = 0.32), or interaction between feather δD values and sex (F = 1.652, df = 1 and 14, P = 0.22). Because Haemoproteus species were not identified until after intensity of infections was determined, we combined both H. elani and H. janovyi in regression analysis of intensity and feather δD values. There was no relationship between intensity of Haemoproteus species and feather δD values (F = 2.277, df = 1 and 24, P = 0.14), sex (F = 0.876, df = 1 and 24, P = 0.36), or interaction between feather δD values and sex (F = 1.158, df = 1 and 24, P = 0.29). Finally, a linear regression analysis was performed, combining intensity of all three hematozoan species detected. There was no relationship between total hematozoan intensity and feather δD values (F = 2.400, df = 1 and 27, P = 0.13), sex (F = 0.988, df = 1 and 27, P = 0.33), or interaction between feather δD values and sex (F = 1.316, df = 1 and 27, P = 0.26; Fig. 3).
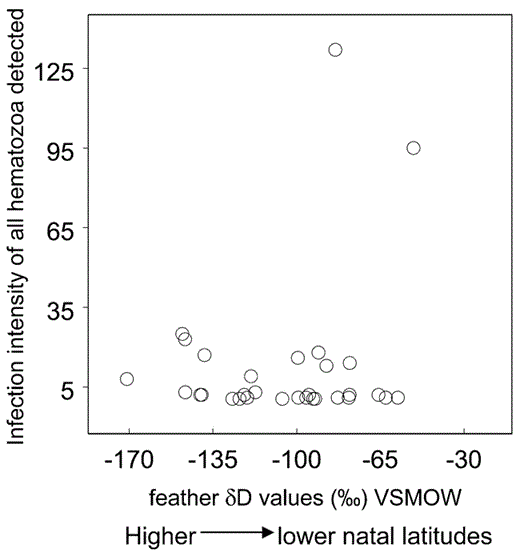
Relationship between feather δD values and intensity of infection of L. toddi and Haemoproteus species detected in hatching-year Sharp-shinned Hawks. Feather δD values were determined using stable-hydrogen isotope analyses (see methods). Total hematozoan intensity was determined by combining calculated intensities of L. toddi and Haemoproteus species for each individual.
Discussion
Distribution of hematozoa
Two genera of hematozoa were found in blood smears from migrating HY Sharp-shinned Hawk samples: Haemoproteus and Leucocytozoon. Haemoproteus species are transmitted by biting midges (Culicoides spp.; Diptera: Ceratopogonidae) and louse flies (Diptera: Hippoboscidae). Because several vector species can carry one to many Haemoproteus species, a specific vector species cannot be singled out as the responsible vector for transmission (Atkinson and van Riper 1991).
The Haemoproteus species identified in our Sharp-shinned Hawk samples were H. elani and H. janovyi. Haemoproteus elani is known to infect a variety of raptor species (Greiner and Kocan 1977, Peirce et al. 1990) and has a wide distribution that includes North America, Europe, Africa, the Middle East, and Asia (Peirce et al. 1990). Haemoproteus janovyi has been found only in vultures of sub-Saharan Africa (Greiner and Mundy 1979).
Leucocytozoon toddi was also found in our samples of Sharp-shinned Hawks. The vectors of L. toddi are black flies (Simulium spp.; Diptera: Simuliidae), which require running water to complete their life cycle. Because Haemoproteus species rely on vectors with more tolerant habitat requirements, they tend to be more widespread than Leucocytozoon species (Greiner et al. 1975, Boal et al. 1998). Our results show that birds infected with H. elani had a wider distribution than those infected with L. toddi (Fig. 1).
Prevalence and migration flyways
Prevalences of Haemoproteus and Leucocytozoon species (37% and 24%, respectively) were slightly higher than those observed by Powers et al. (1994; 28% and 21%, respectively) for HY Sharp-shinned Hawks during fall migration in Pennsylvania. Birds of eastern North America tend to have a relatively lower prevalence of hematozoa than birds of western North America (Greiner et al. 1975). For example, Greiner et al. (1975) found that ∼39% of birds sampled from northeastern North America and 34% of birds sampled from southeastern North America were infected with hematozoa; whereas ∼62% of birds from Alaska south through western Canada and 45% of birds from the western United States were infected with hematozoa. Sharp-shinned Hawks tend to use specific flyways during migration, which reflect their natal or breeding origins (Hoffman et al. 2002). Therefore, hawks migrating through New Mexico are likely of western North American origin (Hoffman et al. 2002, Smith et al. 2003), whereas hawks using the Appalachian flyway in Pennsylvania are probably of eastern North American origin. Therefore, our results support Greiner et al.’s (1975) findings.
Migration and parasites
Studies suggest that birds with a migratory habit are exposed to a larger parasite fauna and thus have a higher prevalence of blood-parasite infections, compared with nonmigratory birds. We found no relationship between natal origins of migrating Sharp-shinned Hawks (feather δD values) and prevalence of infections of L. toddi, H. elani, or H. janovyi (Fig. 2A, B, C). Our data indicate that distance traveled during migration may not affect prevalence of infections. In fact, our results indicate that birds traveling a shorter distance are infected with more parasite species than those traveling greater distances (Figs. 1 and 2D). Some likely reasons for that include: (1) blood-parasite infections may be more prevalent during the breeding season than in autumn; (2) during migration, birds may not stay at stop-over sites long enough to be exposed to vectors; or (3) timing of migration may not coincide with phenology of appropriate vectors. Furthermore, our results may differ from those of other studies because we examined HY birds within a single species. In contrast, other research has investigated multiple species of differing ages and sexes (Bennett and Fallis 1960, Deviche et al. 2001, Figuerola and Green 2000). Parasitemia is known to be affected by age, sex, and phylogenetic relationships (Atkinson and van Riper 1991, Weatherhead and Bennett 1992, McCurdy et al. 1998, Figuerola and Green 2000).
It is often assumed that the energetic cost associated with avian migration is proportional to distance traveled. Therefore, a longer migration may result in fewer resources available to allocate to immune defense (Alerstam 1991). As a result, one might expect not only a higher prevalence but also a higher intensity of parasite infections in birds traveling farther distances. However, we found no relationship between natal origins of migrating Sharp-shinned Hawks (feather δD values) and intensity of infections of Haemoproteus species, L. toddi, or all hematozoan species combined. In fact, the two individuals with highest overall intensity of infection were from relatively lower natal latitudes (Fig. 3). Those results have several possible explanations: (1) birds migrating longer distances may have evolved mechanisms to cope with demands of long-distance travel—for example, Møller and Erritzøe (1998) found that migratory birds have larger immune-defense organs than nonmigratory birds, and Smith et al. (2003) found that female Sharp-shinned Hawks migrating longer distances had higher fat loads; (2) immune responses may vary between individuals or populations; (3) an optimal intensity of hematozoan infection may exist during migration, whereby infections are sustained at an optimal threshold; (4) differential mortality may exist whereby individuals from higher latitudes with heavy parasite loads die before reaching New Mexico—for example, Dawson and Bortolotti (2000) found that American Kestrels (Falco sparverius) infected with hematozoa had a lower return rate to breeding grounds, presumably because of decreased survival during the nonbreeding season; or (5) methods used for assessing parasite prevalence and intensity may have biased our results.
A bird’s age may influence prevalence or intensity of its hematozoan infections (Dawson and Bortolotti 1999) and, consequently, researchers’ ability to detect hematozoa. Sharp-shinned Hawks from lower latitudes typically start breeding earlier in the season than those from higher latitudes (Bildstein and Meyer 2000). In our study, Sharp-shinned Hawks captured earlier in the season tended to originate from lower latitudes, whereas those captured later in the season tended to originate from higher latitudes (Smith et al. 2003). Therefore, we can assume that hawks from both higher and lower latitudes were similar in age when captured and, thus, that the developmental time for hematozoa was also similar.
Studies have found hematozoan infections to have negative effects on body condition of their hosts (Dawson and Bortolotti 2000, Merino et al. 2000, Schrader et al. 2003). To test the relation-ship between body condition and prevalence or intensity of hematozoan infection in migrating Sharp-shinned Hawks, we estimated total body fat of each individual. Fat is the primary source of fuel for migration (Jenni and Jenni-Eiermann 1998) and is commonly used as a proxy for estimating body condition. Body fat was determined using a regression model developed by DeLong and Gessaman (2001) for Sharp-shinned Hawks. We used analysis of variance (ANOVA) to determine the relationship between total body fat and prevalence of infection, and analysis of covariance (ANCOVA) to determine the relationship between total body fat and infection intensity. We found no relationship between total body fat and prevalence of infection (F = 0.253, df = 1 and 71, P = 0.62) or interaction between prevalence and sex (F = 0.066, df = 1 and 71, P = 0.80); however, sex alone was significant (F = 14.460, df = 1 and 71, P < 0.001). We also found no relationship between total body fat and infection intensity (F = 0.178, df = 1 and 27, P = 0.68) or interaction between infection intensity and sex (F = 0.150, df = 1 and 27, P = 0.70); sex, however, was significant (F = 6.553, df = 1 and 27, P = 0.016). Sex was expected to be significant in both tests, because males and females differ in size and total body-fat mass. Our results are similar to those of other studies that have found no influence of hematozoan infections on body mass of birds (Bennett et al. 1988, Bennett et al. 1993).
Biogeography of parasite species
Haemoproteus elani and L. toddi have previously been found in Sharp-shinned Hawks in eastern North America (Kirkpatrick and Lauer 1985, Phalen et al. 1995). Leucocytozoon toddi is a common parasite found in raptors worldwide (Greiner and Kocan 1977).
Our discovery that HY Sharp-shinned Hawks migrating through western North America were infected with H. janovyi is unique. Haemoproteus janovyi has previously been documented only in vultures of southern Africa (Greiner and Mundy 1979). To our knowledge, the present study is the first to record H. janovyi in North America or in Sharp-shinned Hawks. Interestingly, the four individuals infected with H. janovyi and H. elani have natal origins in southwestern North America (Fig. 1).
Because documentation of H. janovyi is absent from studies that have examined hematozoa of Accipitridae from southwestern (Boal et al. 1998), central (Taft et al. 1994, 1996), and eastern (Kirkpatrick and Lauer 1985, Phalen et al. 1995, Powers et al. 1994) North America, it is possibly a new species to the continent. Further confirmation of H. janovyi’s presence in North America requires more-detailed molecular analysis. We found relatively high intensities of parasite infection in individuals infected with H. janovyi, which suggests the response of a “naïve” immune system to a new parasite. If H. janovyi has recently colonized North America, our results imply that southwestern North America may be the original area of colonization. It is also possible that H. janovyi is restricted in North America to the southwestern region.
Acknowledgments
We thank K. Bagnall, W. Beard, S. de la Peña, J. DeLong, L. Greenwood, C. Hisaoka, T. Jacobson, J. Jewell, D. Johnson, W. Lehman, P. McKann, B. Ostheimer, and T. Ostheimer for field assistance. Thanks to the staff of HawkWatch International (HWI) for coordinating research efforts at the Manzano migration research site. We also thank V. Atudorei for dedicated assistance in the stable-isotopes lab and R. DeJong and A. Puetz for help with parasite identification and microscope work. Special thanks to T. Meehan for generating the GIS-based map (http://biology.unm.edu/wolf/precipitationD.htm). T. Meehan, H. Lease, and J. Brown provided thoughtful comments on earlier drafts of the manuscript. Research was funded by the University of New Mexico and the following HWI supporters: U.S. Department of Agriculture, Forest Service, Cibola National Forest; New Mexico Department of Game and Fish; U.S. Fish and Wildlife Service (Region II); National Fish and Wildlife Foundation; Intel Corporation; New Belgium Brewing Company; Baker and McKenzie, Attorneys at Law; Central New Mexico Audubon Society; and HWI members.
Literature Cited