-
PDF
- Split View
-
Views
-
Cite
Cite
Jennifer A. Sheridan, Steven R. Beissinger, Colin R. Hughes, Weak Association Between Measures of Health and Reproductive Success in Green-Rumped Parrotlets (Forpus Passerinus) in Venezuela, The Auk, Volume 121, Issue 3, 1 July 2004, Pages 717–725, https://doi.org/10.1093/auk/121.3.717
- Share Icon Share
Abstract
The relationship between health and demography in the Green-rumped Parrotlet (Forpus passerinus) was examined, using hematological measurements as indicators of health. Variation in adult hematology with gender, age, time during nesting cycle, and breeding status were examined. Only gender and time during nesting cycle showed significant effects on hematology. Females had higher white-blood-cell counts and immunoglobulin-G (IgG) levels than males. Hematocrit was lower during egg laying than at other stages for females, whereas white-blood-cell counts and IgG and alpha-2-macroglobulin (α2m) levels were higher. In males, IgG and α2m levels were higher during egg laying. We expected that hematological indicators of health would predict reproductive success. Some relationships were identified (α2m was negatively related to number of fledglings in males and females, female lymphocytes were negatively related to number of eggs, and basophils were negatively related to number of fledglings) at P < 0.05. However, despite substantial sample sizes (n > 25), none of those relationships were significant after Bonferroni correction, though there appears to be a threshold level for those hematological measures, below which adults may have high or low reproductive success, but above which reproductive success will be low. We suggest that the effect of micro-rather than macroparasites may affect health, resulting in weak effects on reproductive success. Because of the transient nature of microparasitic infection, health and reproductive success may be more significantly related over an organism’s lifetime than in any given year.
RESUMEN
Examinamos la relación entre salud y demografía en Forpus passerinus usando medidas hematológicas como indicadores de salud. Examinamos la variación en la hematología de los adultos en relación al sexo, edad, tiempo durante el ciclo de nidificación y estatus reproductivo. Sólo el sexo y el tiempo durante el ciclo de nidificación mostraron efectos significativos en la hematología. Las hembras presentaron mayores recuentos de glóbulos blancos y niveles de inmunoglobulina-G (IgG) que los machos. El hematocrito fue más bajo para las hembras durante la puesta de huevos que en otras etapas, mientras que los recuentos de glóbulos blancos y los niveles de IgG y alfa-2-macroglobulina (α2m) fueron mayores. En los machos, los niveles de IgG y α2m fueron mayores durante la puesta de huevos. En este estudio esperábamos que los indicadores hematológicos de salud predijeran el éxito reproductivo. Algunas relaciones fueron identificadas (α2m se relaciona negativamente con el número de volantones en machos y hembras, los linfocitos en las hembras están negativamente relacionados con el número de huevos, y los basófilos están negativamente relacionados con el número de volantones) a P < 0.05. Sin embargo, a pesar de un tamaño de muestra substancial (n > 25), ninguna de estas relaciones fue significativa luego de aplicar la corrección de Bonferroni. Sin embargo, parece haber un umbral para estas medidas hematológicas por debajo del cual los adultos pueden tener un éxito reproductivo alto o bajo, pero por arriba del cual el éxito reproductivo será bajo. Sugerimos que el efecto de micro en lugar de macro-parásitos puede afectar la salud, teniendo efectos débiles sobre el éxito reproductivo. Debido al carácter transitorio de las infecciones micro-parasitarias, la salud y el éxito reproductivo pueden estar relacionados más significativamente a lo largo de la vida del organismo que en un año en particular.
Predation, competition, inbreeding depression, disease, stochastic events, and environmental catastrophes can affect population persistence by decreasing reproduction or increasing mortality (Gilpin and Soulé 1986, Lande 1993, Bouzat et al. 1998). Among those, disease warrants particular attention, not only because it can have dramatic effects on reproduction and mortality (Saumier et al. 1986, Møller 1990, Dufva 1996, Merino et al. 2000), but also because its effects are likely magnified by interaction with the other factors. As Lochmiller (1996) pointed out, it may not be highly virulent parasites that are a threat, but rather those normally nonpathenogenic parasites that cause an opportunistic infection when the host's health is compromised by environmental stressors (see also Korschgen et al. 1978).
The growing interest in wildlife disease has led to studies showing that parasites influence demography in numerous ways, some of them indirect. For example, disease is known to cause birds to be more susceptible to predators (Møller and Erritzøe 2000); to affect intrasexual competition by influencing ornamentation (Nolan et al. 1998, Roulin et al. 2001); and to exacerbate the outcome of stochastic events, such as population fluctuations (Soveri et al. 2000). Disease also directly affects population cycles (Hudson et al. 1998, Kukan and Myers 1999, Kamata 2000), reproductive success (Møller 1990, Dufva 1996, Myers 2000) and behavior (Kiesecker and Skelly 2000), and is a major cause of mortality (Thorne and Williams 1988, Roelke-Parker et al. 1996, Hudson et al. 1998).
In birds, effects of disease on reproductive success have received substantial attention. For example, endoparasitic infections are associated with delayed onset of egg laying and production of fewer eggs (Saumier et al. 1986); smaller eggs, proportionally fewer eggs hatching, and smaller nestlings (Dufva 1996); and decreased mean tarsus length, mass, body condition, and survival of nestlings (de Lope et al. 1998, Merino and Potti 1995). Ectoparasite infestations cause a lower proportion of chicks to edge, smaller clutch and brood size, and lower nestling body mass and hematocrit levels (Møller 1990, Richner et al. 1993); whereas medicating against blood parasites results in higher edging success (Merino et al. 2000). The cumulative evidence shows significant relationships between specific parasites and reproductive success in a number of bird species.
The large body of work showing effects of disease on reproductive success is narrowly focused in four ways. First, most studies examine domesticated or captive species (Clubb et al. 1991 a, b, c; Garcia del Campo et al. 1991; D’ Aloia et al. 1995; Melrose et al. 1995; Howlett et al. 1998). Second, the majority of studies examine temperate-region organisms (Saumier et al. 1986, Oppliger et al. 1994, Dufva 1996, Hudson et al. 1998, Møller 1998, Shutler et al. 1999). Third, most studies examine macroparasites (defined by Anderson 1979 as helminthes and arthropods) or larger microparasites, such as hematozoa (Saumier et al. 1986, Møller 1990, Møller et al. 1994, Oppliger et al. 1994, Merino and Potti 1995, Dufva 1996, Shutler et al. 1999, Merino et al. 2000). Fourth, studies examine one or a few diseases at a time (Thorne and Williams 1988, Roelke-Parker et al. 1996, Hudson et al. 1998). That distribution of effort leaves several areas open for study. Much remains to be learned about wild populations, especially those in the tropics, and about effects of viruses and bacteria (microparasites). Also, to understand the full effect of disease on population persistence, the overall effect of disease needs to be assessed.
Here, we report results of a study of Green-rumped Parrotlet (Forpus passerinus) that assessed the effect of disease on reproduction by surveying health of individual birds. Green-rumped Parrotlets provide an ideal system for such a study, because they have large clutches (mean = 7) and substantial variation in clutch size (3–12 eggs), which allows us to examine correlates between clutch size (a measure of reproductive success) and health (using hematological measures). Information on parrot reproduction also has conservation implications, because 30% of Psittaciformes worldwide are threatened with extinction because of the pet trade and habitat loss (Collar and Juniper 1992).
Examining overall health is a direct way of determining effects of disease on reproductive success. An indirect approach would be to study individual diseases and their interactions, and sum across studies; that seems impractical, especially for species where the array of diseases is not known. Only one study has attempted to define the relationship between health and demography in the manner of the present study. Gustafsson et al. (1994) examined the relationship between health and reproductive success in Collared Flycatchers (Ficedula albicollis) and found that increased white-blood-cell, heterophil, and immunoglobulin counts were correlated with decreased clutch size and (along with increased hematocrit) with decreased number of fledglings. They also found positive correlations between hemoglobin concentration and both clutch size and number of fledglings. Therefore, we predicted similar results in our study— specifically, a negative relationship between white-blood-cell counts, immunoglobulin-G (IgG), and alpha-2-macroglobulin (α2m) levels and clutch size and number of fledglings (both measures of reproductive success), and a positive relationship between hematocrit and hemoglobin levels and reproductive success per individual.
Methods
Field methods
We studied a wild population of Green-rumped Parrotlets at Hato Masaguaral (8°34’N, 67°35’W), a ranch in the state of Guárico, Venezuela, between 23 May and 2 December 1999. Green-rumped Parrotlets are small (∼25 g) psittacines that inhabit forest edge, savanna, and pastures in northeastern South America. They breed in cavities, such as tree holes or rotted-out fence posts, and also in nest boxes when provided. Up to 80% of 106 nest boxes designed for the species (Beissinger and Waltman 1991), set up over a 4 km2 stretch of the ranch, were occupied by pairs during the breeding season, which lasts from late May through early December. See Waltman and Beissinger (1992) for further notes on the natural history of the species. Birds were caught in mist nets close to nest boxes and also trapped in those boxes. They were banded (when necessary), weighed, and measured (head length, bill width, radius-ulna length, and wing chord length); a 50 μL blood sample was taken. Body condition was calculated as the residuals of weight regressed on body size (bill width × head length × wing chord, the three most reproducible of the four body measurements taken). Breeding adults were sampled when the first nestling was ∼20 days old. A few birds were also box-trapped during egg laying. Each time a bird was sampled, it was assigned to one of three categories: (1) Prebreeding: any nonbreeding bird or any bird captured before laying eggs; (2) egg laying: birds in the midst of laying a clutch; and (3) day 20: date when first nestling was 20 days old. The prebreeding category is a heterogeneous group, because some birds end up breeding whereas others do not. Birds were also assigned to an age category on the basis of banding year. If a bird was banded as a nestling, its age was known. If it was banded as an adult, it was assumed to be one year old at the time of banding.
Nest boxes were checked every other day for the following: (1) identity of adults at the box, (2) number of eggs laid, (3) number of eggs hatched, and (4) number of chicks fledged. For some nests, the identity of one or both adults was not known because one of the individuals was never spotted or the observer was unable to read the leg bands. Those individuals are referred to as “unknown.“
Hematology
We measured nine variables of the blood sample: (1) hematocrit; (2) hemoglobin concentration; (3) white-blood-cell counts, including quantification of leukocytes—(4) heterophils, (5) lymphocytes, (6) monocytes, and (7) basophils; (8) immunoglobulin-G (IgG); and (9) alpha-2-macroglobulin (α2m). Hematocrit was measured in the field by centrifuging one capillary tube of blood for 5 min and measuring ratio of packed red-cell volume to total volume. Blood from a second capillary tube was expelled onto a glass plate, lysed for 45 s using saponin from a hemolysis applicator, and analyzed using a Leica hemoglobinometer (Leica, Buffalo, New York). For white-cell counts, a smear was made on a slide, fixed in absolute methanol within 3 h, and Giemsa-stained within three weeks in the field. Those smears were labeled and later counted for number (total number in 100 fields at 1,000×) and identity (heterophil, lymphocyte, monocyte, and basophil) of white cells and presence of visible parasites. Serum was preserved 1:9 in SDS buffer and kept cool but not frozen. Upon return to the United States, the equivalent of 1 μL of serum was run on 7% polyacrylamide gel with Tris-glycine running buffer, using chicken (Gallus gallus) IgG and human α2m as internal standards, then stained with Coomassie. For analysis, gel was scanned with a flatbed scanner and analyzed for optical density with ScionImage (Scion Corporation, Frederick, Maryland). Values reported are in milligrams per deciliter (mg dL−1). Because of difficulties with the α2m standards, only the IgG standard was used for calculating amount of protein in each sample. Therefore, α2m values are not comparable with other published values and serve only as a comparison between birds in this data set.
Analyses
Unpaired t-tests (when data were normally distributed) and Kruskall-Wallis tests (when data were non-normal) were used to test for differences between sexes in hematological measures, and ANOVA and Kruskall-Wallis tests were used to determine effects of age and stage in breeding cycle on hematological measures of health. If ANOVA showed an effect of stage or breeding cycle on a hematological measure, paired t-tests were then used to more specifically define the difference between groups. Twotailed P-values are reported.
To examine the relationship between measures of health and reproductive success, reproductive success was defined in two ways: (1) as total number of eggs laid throughout the breeding season, and (2) as total number of nestlings fledged. Although both measures indicate parental fitness, the second is perhaps a slightly better indicator. However, because of predation on some nests, the two measures are not correlated, and sample size of fledged nestlings was smaller. Only nests that fledged at least one chick were used in these analyses to avoid confounding variables, such as predation and failure because of human disturbance. Males and females were analyzed separately to avoid pseudoreplication of reproductive success measures. Health was defined using body condition (residuals of mass on body size: bill width × head length × wing chord, the three most reproducible of the four body measurements taken) and hematological measures. Each of those was used as an explanatory variable in separate regression analyses against the two measures of reproductive success. When more than one sample was taken for an individual, the mean of the measures was used as the explanatory variable, but hematological measures taken during egg laying were omitted, because they differ from those of other stages. In all instances, we used the P < 0.05 significance level.
Results
A total of 223 adults were sampled (137 males and 86 females). There were 123 nesting attempts by 78 identified and 11 unknown males and 82 identified and 12 unknown females. Thirty-nine of those nests were sampled during egg laying, resulting in failure of 14 nests. Thirty-nine nests had at least one chick fledged, with a total of 192 nestlings fledged. Mean, standard deviation, and range of each hematological measure of adult males and females are reported in Table 1. Females have higher white-blood-cell (χ2 = 12.05, df = 1, P < 0.001) counts and IgG levels (χ2 = 10.98, df = 1, P < 0.001) than males. During leukocyte counts, no blood parasites were observed for any individual.
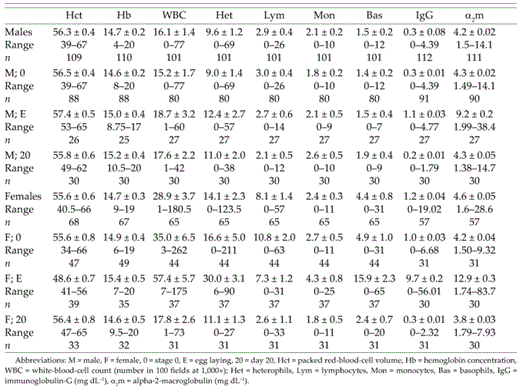
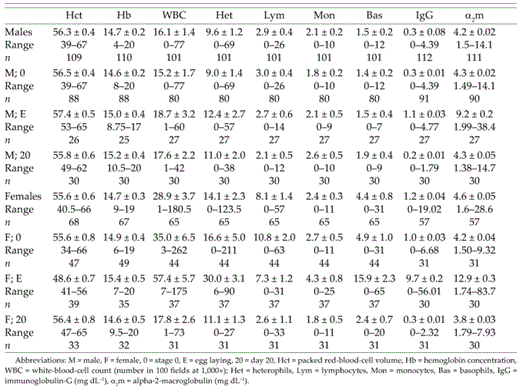
Effects of stage in nesting cycle
Differences in hematological measures between stages of the breeding cycle were analyzed using ANOVA, followed by paired t-tests for hematocrit and Kruskall-Wallis tests for other hematological measures. Because few individuals were sampled at all three stages, intra-individual comparisons of hematocrit were made between pairs of stages. Females have significant differences between stages for hematocrit (F = 27.48, df = 2, P < 0.001), white-blood-cell count (χ2 = 38.50, df = 2, P < 0.001), and both serum protein levels (IgG: χ2 = 52.74, df = 2, P < 0.001; α2m: χ2 = 36.98, df = 2, P < 0.001). Females laying eggs have significantly lower hematocrit than those same females at prebreeding or day 20 (see Table 2 for a summary of post-hoc t-tests) and a peak in white blood cells and serum proteins during egg laying. Males varied significantly between stages for IgG (χ2 = 10.31, df = 2, P = 0.006) and α2m (χ2 = 22.88, df = 2, P < 0.001) with higher values of each during egg laying.
Results of paired t-tests between stages (0 = stage 0; E = egg laying; D20 = day 20) for hematological measures of females.

Results of paired t-tests between stages (0 = stage 0; E = egg laying; D20 = day 20) for hematological measures of females.

Effects of age and comparison of breeders with nonbreeders
Individuals were grouped into age classes: 1 year (n = 57), 2 years (n = 24), 3 years (n = 20), and 4+ years (n = 11) for males; and 1 year (n = 47), 2 years (n = 12), and 3+ years (n = 10) for females. We detected no differences between age classes for any measures in males or females using Kruskall-Wallis tests. Unpaired t-tests comparing nonbreeders with breeders (males: n = 61 and 62; females: n = 17 and 64, respectively) revealed that breeding females had higher IgG than nonbreeders (χ2 = 4.59, df = 1, P = 0.032).
Effects of health on body condition and reproductive success
To examine the relationship between health and body condition and reproductive success, single-factor regression analyses were performed, using each hematological measure and body condition as separate predictive variables, and number of eggs and number of fledglings per individual as the response variables. Number of eggs and number of fledglings per individual were square-root transformed for normality (Shapiro-Wilke test for normality, P > 0.05). Number of fledglings was negatively related to α2m for males (r2 = 0.16, P = 0.04, n = 27). Females showed a similar, though nonsignificant, relationship (r2 = 0.10, P = 0.10, n = 27) as well as a negative relationship between amounts of lymphocytes and number of eggs (r2 = 0.13, P = 0.02, n = 45) and basophils and fledgling number (r2 = 0.11, P = 0.09, n = 27). None of those relationships were significant after sequential Bonferonni corrections. In all those relationships, there appears to be a threshold level below which adults may have high or low reproductive success, but above which low reproductive success will result. For example, when number of fledglings was regressed on male α2m, data points were closely clustered <0.78 and >1.4. Unpaired t-tests showed significant differences in clutch size and number of fledglings between the two groups for all but female α2m (a t-test could not be performed because only one female had very high α2m levels) (see Table 3).
Results of t-tests for reproductive success between groups with very high versus approximately average hematological measures of health.

Results of t-tests for reproductive success between groups with very high versus approximately average hematological measures of health.

Change in hematology may be more informative than any single value, because sick individuals may show declines in health indicators over the nesting period. Therefore, we used regression analyses—with change in each hematological measure from prebreeding to day 20 as the predictive variable and eggs laid and number of fledglings as the response variable— to determine whether those changes predict reproductive success. Although no significant relationships were found in those analyses for either males or females, some high Spearman (r2) values were found in females. Higher numbers of eggs and fledglings were associated with increased hematocrit (r2 = 0.33 and 0.40 for eggs and fledglings, respectively) and hemoglobin (r2 = 0.63 and 0.39), and a decrease in basophils (r2 = 0.45) was associated with higher number of eggs (P > 0.05 in all cases). Lack of significance may be due to the extremely small sample sizes (eight or less in all cases).
Discussion
Female birds are known to show hematological variation over the nesting cycle that can be attributed to stress of breeding and varying activity levels (Schalm et al. 1975). Here, female Green-rumped Parrotlets have lower hematocrit and higher white-blood-cell and serum protein levels during egg laying than at other stages. Similar results have been reported in other studies (Morton 1994, Saino et al. 2001), where females monitored over the course of the breeding season had their absolute lowest hematocrit values during egg laying and immunoglobulin levels peaked just prior to first ovulation. Decreases in hematocrit in parrotlet females could be a result of decreased activity level during egg laying (Schalm et al. 1975); the change in immunoglobulin could result when females alter their immune profile to transmit humoral factors—which provide immune defense against pathogens—to their offspring (Saino et al. 2001). Increased white-blood-cell counts during egg laying are needed to produce increased amounts of IgG (Goulb and Green 1991).
Large variance in hematological indicators of health may make detecting a difference between breeders and nonbreeders easier. If individuals varied little, we might conclude that all individuals are about equally healthy and that health plays little role in determining which birds are breeders and which are nonbreeders. Here, individual values varied widely around the population mean, which possibly suggests differences in health of individuals.
The one prior study using comparable indicators of health and relating those to reproductive success (Gustafsson et al. 1994) found several significant relationships. They found that increased white-blood-cell, heterophil, and immunoglobulin counts were associated with decreased clutch size and (along with increased hematocrit) with decreased number of edglings. They also found positive relationships between hemoglobin concentration and both clutch size and number of fledglings. Here, notably, none of those relationships hold true; but similar, though nonsignificant, relationships were found for other measures of health. High levels of α2m predicted decreased number of fledglings for both males and females, as did basophil counts in females. Also, female lymphocyte count was negatively related to clutch size. In all those relationships, there appears to be a threshold level below which adults may have high or low reproductive success, but above which low reproductive success will result.
Unmeasured sources of variation may obscure the relationship between health and reproductive success. For example, microparasites may be responsible for variation in hematological measures, and those do not affect reproductive success as macroparasites do. Microparasites may cause transient infections, the effects of which are difficult to detect because they tend to be of shorter duration than the effects of macroparasites (Anderson 1979). No previous study reported finding ectoparasites infecting adults in our study population (Beissinger and Stoleson 1991, Waltman and Beissinger 1992, Stoleson and Beissinger 1997, Siegel et al. 1999); though it remains unknown whether endoparasites are present, the absence of eosinophils in the white-blood-cell counts indicates that this population may lack gut parasites. If macroparasites are absent, we would expect to see variation in hematological measures because of microparasites, potentially translating into weak effects on reproductive success. Other studies on the relationship between microparasites and reproductive success in wild populations have failed to demonstrate a connection (Oppliger et al. 1994, Stewart and Rambo 2000), but studies of domesticated poultry have shown decreased fitness. In chickens and turkeys (Meleagris gallopavo), for example, microparasites negatively affect egg production (Cooper and Medina 1999, Dahl et al. 2002). Therefore, it is possible for microparasites to affect reproductive success, but the relationship may be diffcult to detect in wild populations, either because of the transient nature of the infection or because the relationship is weak and obscured by other sources of variation. Although some individuals of the study population of parrotlets showed signs of infection (high white-blood-cell counts and high IgG and α2m levels), the transient nature of many microparasitic infections may limit researchers' ability to detect significant differences in reproductive success associated with those measures.
The present study was completed over one breeding season, so lifetime reproductive success was not measured, and long-term health status is unknown. In a given year, there is likely to be variation in hematological values because of transient infection or dehydration. It is possible that all birds are occasionally infected, but only those that are repeatedly infected show decreased reproductive success. Future studies should examine health measures and reproductive success over successive breeding seasons to address that issue.
Effects of disease will likely increase in severity as populations become smaller (Gilpin and Soulé 1986, Lochmiller 1996), but background data are necessary for such comparisons. Without baseline data on populations' disease status and how that may a affect demography, it will be impossible to say whether or not increased effect of disease is likely to be a major concern. In this population in 1999, we detected no strong relationships between health and reproductive success. However, further studies on other populations are necessary to determine general patterns across taxa with respect to health and demography. We suggest that future studies should encompass several breeding seasons and take experimental approaches. We note that increasing disease load causing decreased reproductive success would be an unsurprising finding. More illuminating would be experimental decreases in disease, leading to increased reproductive success. By manipulating disease load and examining effects on mate choice, nesting attempts, clutch size, and fledgling success, we can further quantify the relationship between health and reproductive success.
Acknowledgments
We thank the University of Miami for funding this project. We are indebted to T. Blohm for his hospitality and for allowing us access to the study site. We also thank B. Sandercock, T. Bruce, D. Seaman, and A. Valcarcel for help in the field. C. Cray and V. Apanius provided expertise with the lab work, and C. Cray also provided invaluable advice in interpreting the results. T. Price and two anonymous reviewers made helpful comments on earlier versions of the manuscript.
Literature Cited