-
PDF
- Split View
-
Views
-
Cite
Cite
Gerald Goldstein, Kelly R. Flory, Beth Ann Browne, Samia Majid, Jann M. Ichida, Edward H. Burtt Jr., Bacterial Degradation of Black and White Feathers, The Auk, Volume 121, Issue 3, 1 July 2004, Pages 656–659, https://doi.org/10.1093/auk/121.3.656
- Share Icon Share
Abstract
When feather-degrading Bacillus licheniformis is grown in culture, it secretes a keratinase that hydrolyzes the β-keratin matrix of a feather, thereby releasing oligopeptides that dissolve into the medium surrounding the feather and feather-degrading bacilli. These peptides absorb light passed through a sample of medium from which feather fragments, melanin granules, and bacteria have been removed by centrifugation. Samples of medium in which white, nonmelanic feathers are degrading absorb more light than samples of medium in which black, melanic feathers are degrading, which indicates that more oligopeptides are dissolved in medium surrounding white feathers than in medium surrounding black feathers. The differential absorption of light supports the conclusion that B. licheniformis degrades white feathers more rapidly than black feathers.
RESUMEN
La bacteria degradadora de plumas Bacillus licheniformis, cuando es criada en cultivo, secreta una queratinasa que hidroliza la matriz de queratina de las plumas, liberando oligopéptidos que se disuelven en el medio que rodea a la pluma y a los bacilos. Estos péptidos absorben luz en una muestra de medio en la que los fragmentos de pluma, los gránulos de me-lanina y las bacterias fueron removidos por centrifugación. Las muestras de medio en que se están degradando plumas blancas sin melanina, absorben más luz que las muestras de medios en que se están degradando plumas negras con melanina, lo cual indica que hay una mayor cantidad de oligopéptidos disueltos en los medios que rodean a las plumas blancas que en los medios que rodean a las plumas negras. La absorción de luz diferencial apoya la conclusión de que B. licheniformis degrada más rápido a las plumas blancas que a las plumas negras.
Dark feathers, which contain melanin, are harder (Bonser and Witter 1993, Bonser 1995) and more resistant to abrasion by airborne particles (Averill 1923; Burtt 1981, 1986) than white feathers, which lack melanin. The feather-degrading bacterium Bacillus licheniformis, isolated from the plumage of birds (Burtt and Ichida 1999), is able to damage white feathers under laboratory conditions that mimic those thought to occur in the plumage (Burtt et al. 2000). Despite the potential for bacterial damage, most flight feathers last from prebasic molt in late summer of one year to prebasic molt in late summer of the next year. Resistance to bacterial degradation may be increased by the presence of melanin, which would harden such feathers against enzymatic action of keratinase secreted by B. licheniformis. We tested that hypothesis by measuring the concentration of oligopeptides—byproducts of β-keratin degradation—in culture flasks of feather-degrading bacilli that contained either black or white feathers.
Methods
We matched length and weight of white secondaries clipped from wings of single comb white leghorn chickens (Gallus gallus domesticus) with length and weight of black secondaries clipped from all-black chickens of similar size in the same flock. Given their physical similarity, we assumed that the black and the white feathers in our samples had similar surface areas exposed to enzymatic action.
We placed 3 g of feathers into 100 mL of feather medium (9.34 mM NH4Cl, 8.55 mM NaCl, 1.72 mM K2HPO4, 2.92 mM KH2PO4, 0.49 mM MgCl2-6H2O, and 0.01% yeast extract [Williams et al. 1990]) in a 250-mL Erlenmeyer flask and sterilized it in a steam sterilizer for 15 min at 121°C. We inoculated each flask with B. licheniformis strain OWU 138B, which was originally isolated from the back of a Willow Flycatcher (Empidonax traillii), according to procedures outlined in Burtt and Ichida (1999). The strain is available from the American Type Culture Collection (ATCC 55768). Inoculated flasks and an uninoculated flask of white feathers were incubated on a gyrorotary incubator at 37°C, rotating at 120 rpm for six days. Rotation aerated the medium, and the uninoculated flask was a control for mechanical damage caused by the rotary action.
Duplicate volumes of 1 mL of medium were removed and centrifuged in sterile microcentrifuge tubes at 10,000 × g to sediment bacteria, feather fragments, and melanin granules. The supernatant solution was placed into quartz cuvettes, and absorbance was measured with a Beckman DU ultraviolet and visible light spectrophotometer. Initially, we measured absorbance at different wavelengths to determine which wavelength was maximally absorbed. Then we used the maximally absorbed wavelength to compare absorbance of the medium in which black feathers were degrading with absorbance of the medium in which white feathers were degrading.
We also measured serial dilutions of known concentrations of bovine serum albumin. We calculated absorbance measurements of unknown concentrations of oligopeptide fragments from degraded feathers by comparing them to absorbance measurements of known concentrations of pure protein in the bovine serum albumin samples.
Results
Oligopeptide fragments of β-keratin were released as a byproduct of feather degradation by B. licheniformis. Such fragments were not visible, but had an absorption spectrum that peaked at 230 nm (Fig. 1). Furthermore, absorbance measurements of serial dilutions of bovine serum albumin (Fig. 2) indicated that absorbance measurements of 0–2.2 at 230 nm over a range of 0–500 μg mL−1 were directly proportional to protein concentration. Therefore, absorbance of a sample of supernatant fluid from the liquid medium that surrounds a degrading feather determined the concentration of oligopeptides in solution, which was a function of the rate of feather degradation.

Absorption spectrum of oligopeptide fragments produced by bacterial degradation of β-keratin. Maximum absorbance occurs at 230 nm.
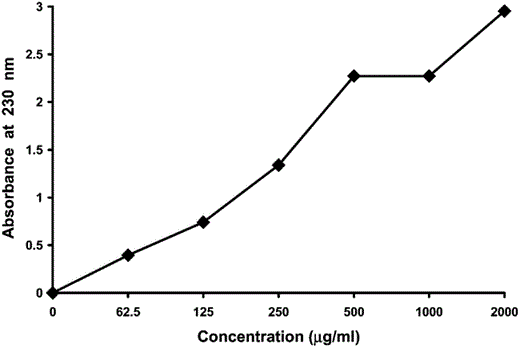
Absorbance of different concentrations of bovine serum albumin at 230 nm.
Within 42 h after feathers were inoculated with feather-degrading bacilli, oligopeptides were present in the liquid medium surrounding the feathers (Fig. 3). Thereafter, concentration of oligopeptides, as measured by absorbance of the medium, increased rapidly in samples drawn from medium surrounding white feathers (Fig. 3). In contrast, absorbance hardly changed in samples drawn from medium containing black feathers (Fig. 3).

Absorption of radiation at 230 nm by media containing dissolved oligopeptides released by bacterial degradation of white (open triangles, dashed line) or black (solid boxes, solid line) secondary feathers from leghorn chickens.
Discussion
The difference in absorbance at 230 nm can only result from a difference in the concentration of suspended oligopeptide fragments. That difference indicates a difference in the rate of degradation by the keratinase enzyme. Black feathers degraded slowly or not at all, whereas white feathers degraded rapidly.
Presence of melanin in feathers increases their resistance to abrasion (Burtt 1981, 1986; Bonser 1995) and bacterial degradation; multiple mechanisms acting in concert probably account for the effect. Melanin is a granular protein that is distributed throughout the β-keratin matrix of the cortex (i.e. outer layer) of the rachis and barbs of black or brown feathers. Thus, a black or brown cortex is a composite material, and such materials are known to be harder (Bonser and Witter 1993) than noncomposites, such as the nonmelanic β-keratin that forms the cortex of white feathers.
Voitkevich (1966) showed that the cortex of melanic barbs and rachises is thicker than the cortex of nonmelanic barbs and rachises. A thicker cortex might enable melanic feathers to withstand greater damage from abrasion and bacterial degradation before breaking, but a thicker cortex cannot account for the lower concentration of oligopeptide fragments in medium containing melanic feathers than in medium containing nonmelanic feathers. That difference suggests that the keratinase enzyme is less successful in hydrolyzing β-keratin when granules of melanin are embedded in the matrix than when they are absent.
Unlike α-keratin, which has a relatively simple helical structure, β-keratin has a complex structure in the form of a pleated sheet (Pauling and Corey 1951, Brush 1978) with disulfide bonds connecting branches of the same and different molecules. Presence of melanin granules during keratinization of the developing feather may provide additional surface, which would bring sulfur atoms close together and thereby catalyze bonding of sulfur atoms within and between molecules of β-keratin. If the active site of the enzyme could not accommodate the additional disulfide bridges, degradation of β-keratin would be greatly slowed.
Another possibility is that melanin affects the bacterium directly. The evidence is circumstantial; but in brown trout (Salmo trutta), the skin surrounding the site of a bacterial infection becomes melanized, which inhibits the spread of the infection (Burkhardt-Holm et al. 1997). The direct cause of the inhibition is unknown, but it may be that melanin inhibits enzymatic activity, thereby decreasing the rate of bacterial degradation of dark feathers.
Although we do not understand how melanin protects feathers from degradation by B. licheniformis, we have shown that it does. Feathers are subject to many different selection pressures (Burtt 1986, Burtt and Ichida 2004a). Melanin, which provides a range of colors from gray to black, buff to red-brown and brown, provides camouflage for many birds and display colors for others. Its presence increases the abrasion resistance of feathers, which is important to flight feathers and accounts for the dark color of primaries. We have shown that the presence of melanin increases feathers' resistance to bacterial degradation by the ubiquitous soil bacterium B. licheniformis, which is also commonly found in the plumage of birds. Such resistance may be important to birds that live in humid environments where bacteria are abundant and active. Burtt and Ichida (2004b) have shown that dark Song Sparrows (Melospiza melodia morphna) from the Pacific northwest, where relative humidity is high, carry strains of B. licheniformis in their plumage that degrade feathers more rapidly and completely than strains of B. licheniformis carried in the plumage of pale Song Sparrows (M. m. fallax) from southeastern Arizona, where relative humidity is low. The pattern of dark plumage where relative humidity is high and pale plumage where it is low was first described by Gloger (1833) and can be seen in many wide-ranging species (Zink and Remsen 1986). We suggest that, in addition to its other functions, an important selective advantage of melanic plumage is its resistance to degradation by bacteria that are part of every bird's environment.
Acknowledgments
We thank P. Flattery for allowing us to cut the secondaries from her chickens. We thank T. C. Grubb, Jr., and an anonymous referee for their suggestions on an earlier draft. Our work was supported by the National Science Foundation (BIR-998805) and by the Leland and Helen Schubert Endowment in support of the Honors Program at Ohio Wesleyan University.
Literature Cited