-
PDF
- Split View
-
Views
-
Cite
Cite
Mikaela Kislevitz, Karen B Lu, Christine E Wamsley, Shyon Parsa, John E Hoopman, Jeffrey M Kenkel, Yucel Akgul, Bipolar Fractional Radiofrequency Treatment of Suprapatellar Skin Assessment Using Noninvasive Devices and Microbiopsy, Aesthetic Surgery Journal, Volume 41, Issue 12, December 2021, Pages NP1997–NP2008, https://doi.org/10.1093/asj/sjab210
- Share Icon Share
Abstract
Noninvasive treatments for skin tightening are gaining popularity. However, no studies have assessed bipolar fractional radiofrequency treatment on suprapatellar skin.
The purpose of this study was to evaluate the efficacy of bipolar fractional radiofrequency treatment on suprapatellar skin.
Twenty patients received 1 bilateral suprapatellar bipolar fractional radiofrequency treatment. They returned 7 days, 3 weeks, 3 months, and 6 months posttreatment. Noninvasive measurements were obtained at each visit, including high-resolution ultrasonography, optical coherence tomography, transepidermal water loss and skin elasticity. Microbiopsies were collected in the treatment area for histologic and gene expression analyses. Three clinicians completed photographic evaluations comparing texture and laxity at baseline vs 6 months posttreatment.
Fifteen subjects completed all 6 visits. Both transepidermal water loss and dermal-epidermal junction roughness were increased at 3 weeks and 3 months posttreatment. Both attenuation coefficient and stiffness were increased significantly at 3 and 6 months posttreatment. Blood flow 0.5 mm below the surface and expression of epidermal hyaluronic acid and inflammatory genes IL-1b and IL-6 were significantly higher at 7 days posttreatment compared with pretreatment and 3 months posttreatment. There were no statistically significant changes in collagen- or elastin-related genes and proteins at 7 days or 3 months posttreatment. An improvement in texture and laxity was observed at 6 months posttreatment in 17.7% and 24.4% of photographs, respectively.
Radiofrequency treatment by microneedling of suprapatellar skin shows limited effects in decreasing skin laxity and improving skin appearance. At a molecular level, the treatment resulted in lower elastin and hyaluronic acid levels and increased dermal-epidermal junction roughness based on histology and optical coherence tomography imaging.
The use of minimally invasive skin rejuvenation treatments has been gaining in popularity in recent years. Several of these energy-based devices, including laser, radiofrequency, infrared, and ultrasound, have been targeted towards improving facial skin appearance. The use of these devices has grown to include improving the appearances of other body parts such as the arms, abdomen, and thighs. Of these devices, microfocused ultrasound has been the preferred method for off-face regions but has shown limited success in tightening and reducing laxity of the knee region and suprapatellar skin.1,2
Bipolar radiofrequency microneedles have shown success in reducing facial rhytids and skin laxity of the lower facial and jowls.3 The suprapatellar skin of the knee possesses similar qualities to the skin in the lower face and upper neck. Though FDA-approved for the treatment of facial wrinkles and the treatment of cellulite, it is off-label for use in the knee. Currently, no devices are specifically indicated to treat laxity in the knee region. Based on its known effects on facial skin, it can be hypothesized that bipolar fractional radiofrequency will cause similar results in suprapatellar skin, lifting and reducing laxity of the skin in that region.
Like many of the devices currently on the market, bipolar radiofrequency microneedling devices promote neocollagenesis and neoelastinosis by inducing controlled thermal damage in the dermis.4 Radiofrequency devices, unlike lasers, are chromophore-independent, provide better penetration than lasers, and spare sweat glands, sebaceous glands, and hair follicles.3,5
Several studies have shown bipolar radiofrequency microneedles to be safe and effective as a noninvasive skin rejuvenation treatment for lax facial tissue.5-9 The purpose of current study was to evaluate the safety and efficacy of bipolar fractional radiofrequency treatment for tightening and improving laxity of the suprapatellar skin.
METHODS
Study Approval and Skin Treatments
The study protocol was approved by the University of Texas Southwestern Medical Center IRB; study procedures were performed between April 2018 and February 2019. Patients consented to the treatment protocol, including digital photography, prior to treatment. Twenty women between the ages of 18 and 75 years with Fitzpatrick skin types I to III received a single treatment with the bipolar fractional radiofrequency microneedling device (Profound System; Candela Corporation, Wayland, MA) on the suprapatellar skin of both the left and right legs. All subjects were injected with local anesthesia consisting of 1% to 2% lidocaine with 1:100,000 epinephrine. Exclusion criteria included individuals who had undergone dermatologic procedures for the treatment of wrinkles, skin resurfacing, or skin rejuvenation in the treatment area within 1 year of study participation.
Bipolar Fractional Radiofrequency Microneedling Device
The Profound System is an FDA-cleared device, with 510(k) approval, for percutaneous treatment of facial wrinkles and improvement in the appearance of cellulite in patients. The device consists of a console containing a radiofrequency generator and a graphic user interface to adjust treatment parameters, 2 reusable treatment applicators, and 2 disposable, single-use, sterile electrode cartridges. Specifically, the 25° dermal applicator and cartridge are used for the treatment of facial wrinkles and were used to treat the suprapatellar skin in this study. The 25° applicator has 5 independent, paired channels (10 needles) and covers a treatment area of 14 mm at a depth of 1 to 2 mm. The radiofrequency component of the Profound System delivers 460 ± 5 kHz. Bipolar radiofrequency radiation travels from the radiofrequency generator, through the electrodes, into the dermal layers beneath the surface of the skin. On the left and right suprapatellar regions, approximately 132 [21] insertions (mean [standard deviation]) and 131 [22] insertions were completed during the treatment, respectively.
Noninvasive Devices
AquaFlux AF200-01 (BioX Systems Ltd, London, UK)
To evaluate the barrier function of the skin, transepidermal water loss (TEWL) was measured approximately 3 to 4 cm above the center of each patella prior to treatment, and at 7 days, 3 weeks, 3 months, and 6 months posttreatment.
VivoSight (Michelson Diagnostics, Maidstone, UK)
A VivoSight optical coherence tomography (OCT) device was used to acquire skin images at 1305-nm laser center wavelength, creating a 6 mm × 6 cm 250-frame real-time image of facial skin 1 to 2 mm under the skin surface. The VivoSight probe was placed 3 to 4 cm above the center of each patella to acquire images prior to treatment, and at 7 days, 3 weeks, 3 months, and 6 months posttreatment. Vertical B-scan, en-face and 3-dimensional images were produced in order to calculate vasculature density, surface and dermal-epidermal junction (DEJ) roughness, and the optical attenuation coefficient, a measure of light absorption and scattering.
DUB SkinScanner75 (taberna pro medicum GmbH, Lüneburg, Germany)
High-resolution ultrasonography images were taken by placing the probe 90° to the skin surface 3 to 4 cm above the center of each patella to acquire images prior to treatment, and at 7 days, 3 weeks, 3 months, and 6 months posttreatment. The measurements were begun at 250% amplification and 4-mm penetration depth with a 75-MHz applicator. Measurements were taken at 5, 6.4, 8, 10, 13, and 16 mm.
BTC-2000 (SRLI, Nashville, TN)
The BTC-2000 suction device utilizes an infrared laser to measure skin elasticity parameters within a vacuum-sealed environment. The vacuum aperture was placed 90° to the skin surface, 3 to 4 cm above the center of each patella, and attached to the skin with a double-sided adhesive disc. The pressure within the chamber was raised to 150 mmHg, maintained for 3 seconds, and then released for a 3-second relaxation period. Elastic deformation, viscoelastic deformation, ultimate deformation, energy absorption, elasticity, and stiffness were recorded. Elastic deformation (mm) is the amount of deformation occurring at the point of maximum pressure. Viscoelastic deformation (mm) is the time-dependent, viscous deformation of tissue caused by a constant application of pressure. Ultimate deformation (mm) is the sum of elastic and viscoelastic deformation. Energy absorption (mmHg × mm) is a measure of overall tissue compliance; higher energy reflects a softer, more compliant material. Elasticity (mm) is the amount of elastic recovery, or reverse deformation, that occurs upon the release of pressure. Stiffness (mmHg/mm) is the mechanical behavior of the structure; several factors account for this property, including material shape, size, and content.10 Measurements were obtained prior to treatment, and at 7 days, 3 weeks, 3 months, and 6 months posttreatment. The settings included: maximum pressure = 150 mmHg, time period for relaxation = 3 seconds, number of cycles = 1, data sample rate = 10 Hz, linear negative pressure rate = 10 mmHg/s, test chamber size = 10 mm. The BTC-2000 has been used in a number of studies to assess biomechanical skin properties.11-13
Microbiopsy
Collection
Core skin biopsies were extracted with microbiopsy punches (0.33-mm inner diameter, 5 mm long, catalog number YA0016; World Precision Instruments, Sarasota, FL) prior to radiofrequency treatment and at 7 days and 3 months posttreatment. The core biopsy samples were taken 3 to 4 cm above the center of the patella on the left leg to perform both histologic and gene expression assessments.
Immunostaining
Following collection, microbiopsies were immediately fixed in 4% paraformaldehyde (Sigma-Aldrich, St Louis, MO) for 4 hours, then kept in 50% ethanol until paraffin embedding. Immunostainings were done as described previously.14 Briefly, sections (5 μm thick) were deparaffinized and blocked with 10% normal goat serum (Life Technologies Corporation, Carlsbad, CA) for 20 minutes at room temperature. Subsequently, tissue sections were incubated with primary antibody, biotinylated hyaluronic acid–binding protein (HKD-BC41; Cosmobio, Carlsbad, CA), Collagen I (ab6308; Abcam, Cambridge, MA), Collagen III (ab7778; Abcam), elastin (ab2160; Abcam), Ki67 (catalog number VP-K452; Vector Laboratories, Burlingame, CA) for approximately 16 hours at 4°C. The slides were washed 3 times with phosphate-buffered saline, then incubated with a solution containing fluorescein-tagged streptavidin (1:500 dilution), or Alexa Fluor 488– or 555–conjugated secondary antibodies (Ig, heavy and light chains; 1:1000 dilution; Life Technologies Corporation) in 10% normal goat serum. This secondary incubation was done for 1 hour at 25°C. Slides were washed 3 times in phosphate-buffered saline, and coverslips were mounted to the slides with Prolong Gold containing 4′,6-diamidino-2-phenylindole (Life Technologies Corporation). Collagen and elastin sections were subject to antigen retrieval by boiling in sodium citrate buffer (10 mM, pH 6) for 20 minutes prior to blocking. Terminal deoxynucleotidyl transferase dUTP nick end labeling (TUNEL) staining for apoptotic cells was done with paraffin sections (5 μm thick) according to the protocol supplied with the Promega DeadEnd Fluorometric TUNEL System (Promega Corporation, Madison, WI). Apoptotic cells were labeled with fluorescein, and sections were counterstained with propidium iodide. Images were obtained with a Zeiss laser scanning confocal microscope (Zeiss, Jena, Germany). ImageJ software was used to generate individual images. Paraffin processing, embedding, sectioning, and histologic staining with hematoxylin-eosin and Masson trichrome were performed as described previously.14
RNA Isolation, Amplification and Quantitative Real-Time Polymerase Chain Reaction
Total RNA was extracted with a microRNeasy kit (Qiagen, Germantown, MD) in 14 μl nuclease-free water and treated with DNase-I (DNA-free; Ambion Inc., Austin, TX). cDNA synthesis was performed with 1 μl of total RNA volume iScript Reverse Transcription Supermix followed by SSO Advanced PreAmp supermix according to the manufacturer’s protocol (Bio-Rad Laboratories, Inc., Plano, TX). Quantitative real-time polymerase chain reaction was performed with Taqman primers and a PRISM 7900HT Sequence Detection System (Applied Biosystems, Waltham, MA). Aliquots of amplified cDNA were used for each reaction and were run in triplicate. Each gene was normalized to the expression of the housekeeping gene human ActinB. Gene expression was calculated according to the 2–ΔΔCt method, as described by the manufacturer (user bulletin number 2; Applied Biosystems).
Photography and Evaluations
Subjects were photographed at baseline, and at 7 days, 3 weeks, 3 months, and 6 months posttreatment according to the following procedures. Standard and close-up photographs of the suprapatellar region of both legs were taken for evaluation of skin texture and laxity. Unblinded assessment was performed by 3 clinicians (one plastic surgery fellow, one plastic surgery resident, one medical student) based on photographs of subjects taken prior to the first treatment and at 6 months posttreatment. The reviewers were asked to determine whether they believed the photograph taken 6 months posttreatment showed an improvement, no change, or worsening in skin texture and skin laxity compared with baseline.
Statistical Analysis
Statistical analysis was performed with GraphPad Prism version 7 (GraphPad Inc., San Diego, CA). The per-protocol population included all subjects who received treatment and attended the 4 follow-up visits in order to complete the study in accordance with the protocol. Paired t tests were used to evaluate the percentage change from baseline (before treatment or untreated skin) for data acquired from noninvasive skin assessments at 7 days, 3 weeks, 3 months, and 6 months posttreatment. One-sample t tests were used to evaluate biopsy results from samples extracted at baseline, 7 days, and 3 months posttreatment. Statistical significance was determined by a P < 0.05.
RESULTS
Study subjects ranged from 24 to 58 years of age, with a mean [standard deviation] age of 52.9 [9.19] years. Of the 20 subjects enrolled, 15 completed all 6 study visits. Figures 1 and 2 illustrate 2 representative photographic series pretreatment, and at 3 and 6 months posttreatment.
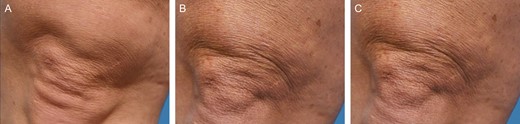
Representative clinical photography series of a 46-year-old female subject at baseline (A), 3 months posttreatment (B), and 6 months posttreatment (C).
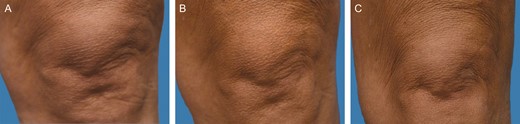
Representative clinical photography series of a 44-year-old female subject at baseline (A), 3 months posttreatment (B), and 6 months posttreatment (C).
Changes in the skin texture and laxity of the right and left suprapatellar region were assessed by 3 clinicians based on photographs taken pretreatment and at 6 months posttreatment (Figure 3). There was no change in the texture and laxity of the left and right suprapatellar skin based on the assessment of the 3 reviewers. On average, the reviewers reported an improvement in texture in 26.7% and 8.9% of the left and right suprapatellar posttreatment photographs, respectively. Laxity appeared improved, on average, in 17.8% and 31.1% of the left and right suprapatellar posttreatment photographs, respectively. Both texture and laxity appeared worsened posttreatment in 28.9% of the left suprapatellar skin. In the right suprapatellar skin, the reviewers reported a worsening of texture and laxity in 31.1% and 15.6% of the posttreatment photographs, respectively.
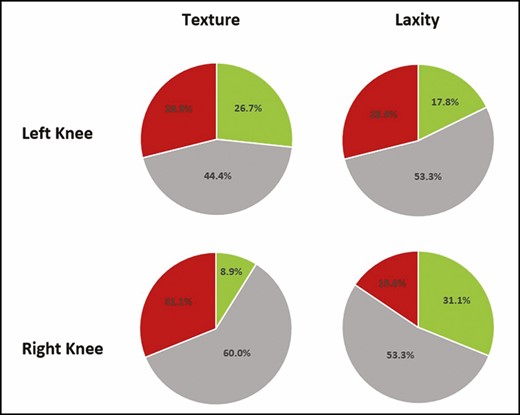
Results of unblinded photographic evaluations by 3 clinicians to assess changes in texture and laxity at 6 months posttreatment compared with baseline (pretreatment). Green, improvement; red, worsened; gray, no change.
TEWL increased 17.8% (P = 0.004) and 25.3% (P = 0.0493) at 3 weeks and 3 months posttreatment compared with baseline (Figure 4). Although the 11.1% increase in epidermal thickness was significant at 7 days posttreatment (P = 0.0079), all other measurements of epidermal thickness posttreatment did not show any statistically significant changes compared with baseline (Figure 5A,B). This result was consistent with the histologic analysis of the epidermal layer thickness (data not shown). As shown in Figure 5A,C, dermal thickness, assessed by the DUB SkinScanner75, at 3 weeks posttreatment was increased 14.7% compared with baseline (P = 0.0013). Changes in dermal thickness were not significant at 3 and 6 months posttreatment (Figure 5C). The dermal epidermal junction roughness (epidermal ridges), based on Ra calculation, increased 50% at 3 weeks and 3 months and 20% at 6 months posttreatment (Figure 6A,B).
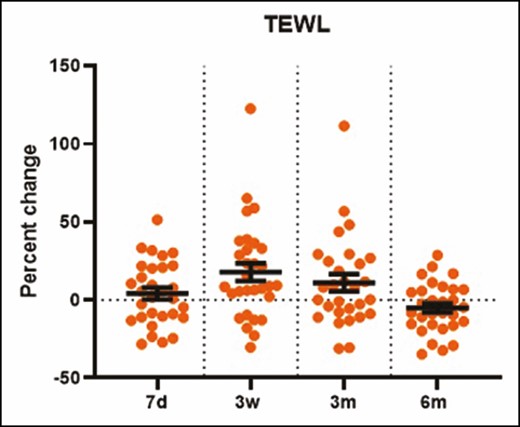
The percentage change in transepidermal water loss (from AquaFlux measurements) increased significantly at 3 weeks posttreatment (P = 0.004). d, day; m, month; w, week.
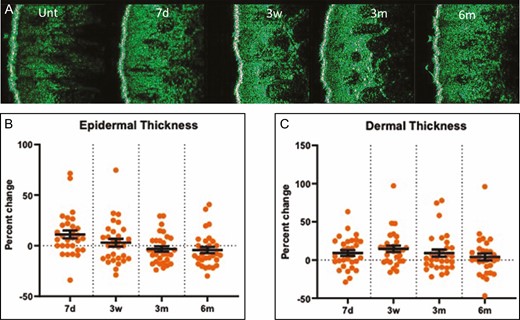
Dermal and epidermal thickness measured by DUB SkinScanner75 images (A). The percentage temporal change in epidermal thickness (B) and dermal thickness (C) as measured by the DUB SkinScanner75. Each dot on the graph represents a single patient at a given time point. d, day; m, month; unt, untreated; w, week.
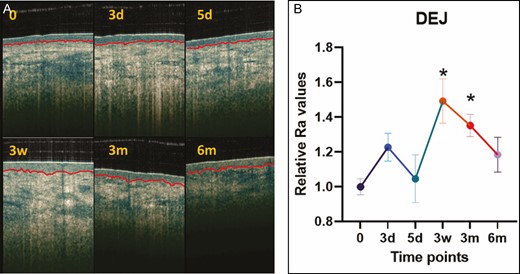
The dermal-epidermal junction assessed from optical coherence tomography images (A) and charted as relative Ra value demonstrating the roughness at 7 days, 3 weeks, 3 months, and 6 months posttreatment (B). *P < 0.05 vs untreated skin or baseline. d, day; m, month; w, week.
The radiofrequency treatment resulted in an increase from baseline in the dermal attenuation coefficient of 18.7% at 3 months following treatment (P < 0.0001) and 30.3% at 6 months following treatment (P < 0.0001) (Figure 7). The BTC-2000 measurement of stiffness was increased 9.08% (P = 0.023) and 8.66% (P = 0.0119) at 3 and 6 months posttreatment, respectively (Figure 7). However, the skin elasticity and laxity did not alter after the treatment. There was a wide variation in the percentage change of laxity with no statistical significance compared with baseline among subjects (Figure 8).
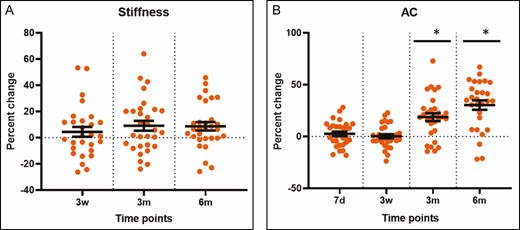
(A, B) Temporal changes in the attenuation coefficient and stiffness measured by optical coherence tomography and with the BTC-2000 device, respectively, at 7 days, 3 weeks, 3 months, and 6 months. Each dot in the graphs represents a single patient at a given time point. *P < 0.05 vs untreated skin or baseline. AC, attenuation coefficient; d, day; m, month; w, week.
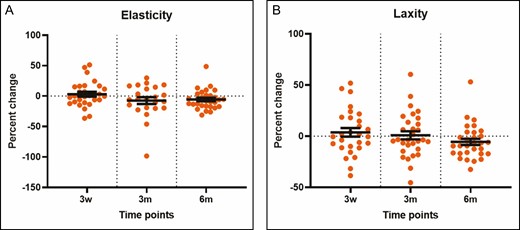
(A, B) Temporal changes in the elasticity and laxity measured by the BTC-2000 device at 7 days, 3 weeks, 3 months, and 6 months. Each dot in the graphs represents a single patient at a given time point. *P < 0.05 vs untreated skin or baseline. m, month; w, week.
The immunostaining of the microbiopsy sections revealed that the collagen I and III abundance in dermis did not change significantly following treatment (Figure 9). However, both elastin and hyaluronic acid (Figure 10) staining demonstrated a significant decrease at 3 months following treatment, declining 55% and 46%, respectively. However, there was no change in gene expression of elastin, collagen I, collagen III, and crosslinking factor lysyl oxidase following treatment compared with untreated baseline (Figure 11).
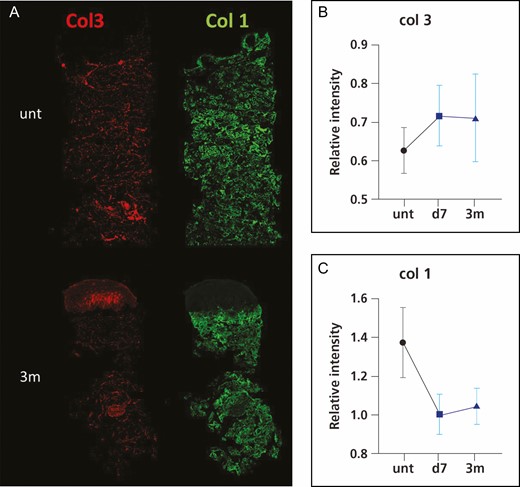
Representative immunofluorescence images of collagen III (col3) and collagen I (col1) (A) and intensity quantification of collagen III (B) and collagen I (C). *P < 0.05 vs untreated skin or baseline. d, day; m, month; unt, untreated; w, week.
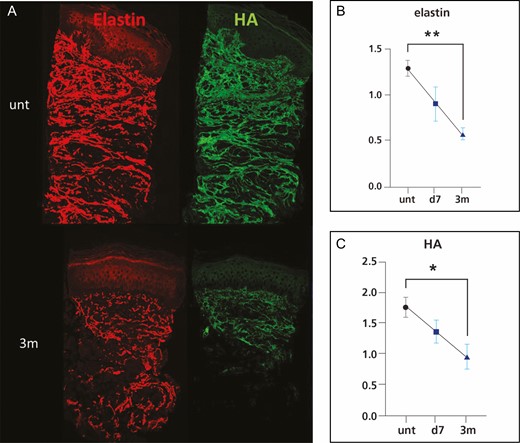
Representative immunofluorescence images of elastin and HA (A) and intensity quantification of elastin (B) and HA (C). *P < 0.05 vs untreated skin or baseline. d, day; m, month; unt, untreated, HA, hyaluronic acid.
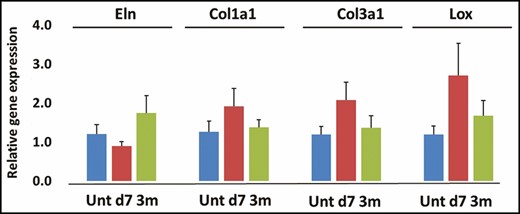
Gene expression of elastin (Eln) (A), collagen I (Col1a1) (B), collagen III (col3a1) (C) and lysyl oxidase (Lox) (D). d, day; m, month; unt, untreated.
Gene expression of inflammatory factors tumor necrosis factor α (TNFα) and interleukin 6 (IL-6) increased significantly 7 days following treatment, without change in inflammatory cell expression based on hematoxylin-eosin staining of the 0.33-mm microbiopsies extracted from the knee at baseline, 7 days, and 3 months posttreatment (Figure 12).
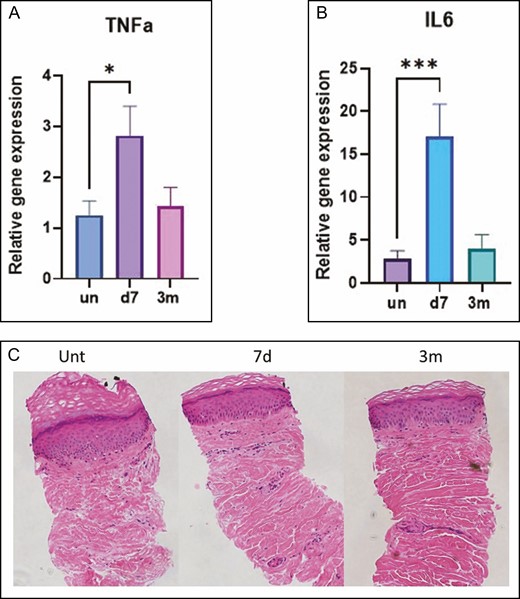
(A-C) Gene expression of the inflammatory markers TNFα and IL-6. Hemotoxylin-eosin staining of microbiopsy sections at baseline (untreated), and at 7 days and 3 months posttreatment. *P < 0.05, ***P < 0.001 vs untreated skin or baseline. d, day; m, month; unt, untreated; IL, interleukin; TNFα, tumor necrosis factor α.
As shown in Figure 13, significant changes in vasculature were noted with the OCT device throughout the posttreatment time course. Blood flow 0.5 mm below the surface increased 8.2% from baseline at 7 days posttreatment. However, blood flow at this depth decreased 58.5% and 86.9% at 3 and 6 months posttreatment, respectively. Blood flow at 0.25 mm had also increased 18.5% from baseline at 7 days posttreatment.
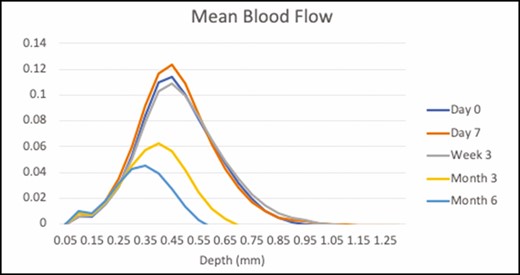
Mean blood flow assessed by optical coherence tomography at baseline (blue), and at 7 days (orange), 3 weeks (gray), 3 months (yellow), and 6 months (green) posttreatment.
DISCUSSION
Less-invasive, nonsurgical treatments—and in particular, devices to tighten skin and decrease laxity—are gaining popularity. These options offer the appeal of minimized recovery time and may ease concerns over potential surgical complications. Most of these devices, including bipolar fractional radiofrequency devices, are typically utilized on facial skin.3,5,8,15 Little peer-reviewed data exist on the use of these devices in areas off of the face. Little investigation has been performed of bipolar fractional radiofrequency in combination with microneedling to treat focal areas of excess skin adiposity, such as the upper arm, upper back, and abdomen.16 Additionally, 1 pilot study examined the potential use of fractional microneedle radiofrequency for primary axillary hyperhidrosis.17 Although the use of fractional bipolar radiofrequency with microneedling has also been assessed for the treatment of cellulite and skin laxity of the upper leg, these studies have typically been evaluated subjectively, mainly through patient satisfaction surveys and clinician or observer improvement rating scales.18,19 In reviewing the literature, no studies have been published objectively investigating this treatment on the suprapatellar region.
By means of noninvasive skin evaluation tools and scarless 0.33-mm microbiopsy, here we have evaluated the efficacy of a bipolar fractional radiofrequency device on knee skin. Although there was no change in the subjective evaluation of the knees based on photograph and patient surveys, the microbiopsy and noninvasive tools showed a significant increase in DEJ roughness, TEWL, dermal attenuation coefficient, as well as a decrease in elastin and hyaluronic acid abundance and blood flow following a single bipolar radiofrequency treatment.
The current study demonstrated that a single bipolar radiofrequency treatment had no effect on suprapatellar skin appearance and epidermal thickness (Figures 1, 2, 3, and 5), suggesting no structural changes related to epidermis. Dermal thickness and biomechanics (laxity, elasticity, and stiffness) were not altered by the treatment (Figures 5, 7, and 8), suggesting no effect of the treatment on dermal function either. Our findings differ from previous studies showing that significant improvements in facial skin wrinkles and texture can be achieved with radiofrequency devices,20,21 as well as arm and abdominal skin laxity.5-9,22 In addition to variations in the strength of the radiofrequency treatment regimens between studies, this discrepancy may be due to structural and functional differences in anatomic areas of skin: recent studies have documented that different anatomic locations of skin treatment may yield different outcomes.23-25 For example, Bailey et al found that a fractional CO2 treatment on facial tissue resulted in an attenuation of laser energy and significantly shorter laser column depths compared with an abdominal treatment, likely due to a divergence of the laser energy around pilosebaceous units and a lateral spread of energy along blood vessels.23 These significant anatomic and biophysical differences, such as concentration of pilosebaceous units, concentration of sebum, moisture content, dermal vascularity, and skin thickness, may, therefore, affect the degree of treatment penetration and distribution of energy.23,24 Additionally, suprapatellar skin does not have a tremendous amount of underlying subcutaneous tissue. It is our belief that dermal application of heat does impact the underlying fat and can result in improvement in contour and even skin tightening.16,26,27
Although the current study failed to document significant clinical outcomes, there were alterations in the skin at the ultrastructural level following treatment. The DEJ became rougher at 3 weeks and 3 months, flattening closer to basal levels at 6 months (Figure 6). This suggests a temporary subepidermal remodeling/rejuvenation with increased epidermal permeability following the treatment. With aging, thinning of the epidermis and effacement of the rete ridges result in flattening of the DEJ, a process that contributes to wrinkles and epidermal laxity.28 However, skin biomechanics did not detect changes, including stiffness, laxity, or elasticity, in the current study (Figures 7 and 8).
In agreement with the changes in DEJ, the TEWL increased at 3 weeks and 3 months following treatment (Figure 4). This could indicate a transient decrease in skin barrier function. No statistically significant change was noted at 6 months posttreatment, which may suggest a return to normal barrier function over time. Measurements of TEWL have been utilized to gain a better understanding of skin barrier function, particularly in a number of dermatologic studies related to disease processes and healing.29,30
In addition, the attenuation coefficient measured by OCT to show dermal density documented significant increases at 3 and 6 months (Figure 7), with no change in collagen abundance (Figure 9). This appears to be due to a decrease in other extracellular matrix molecules, mainly hyaluronic acid and elastin (Figure 10), and vasculature/blood flow (Figure 13). However, there was no change in the gene expression of extracellular matrix molecules (Figure 11). The optical attenuation coefficient is a measurement that indicates the amount of light absorbed or reflected in tissue.31,32 Specifically, denser tissues scatter a greater deal of light and will therefore have a higher optical attenuation coefficient.33 Thus, a higher attenuation coefficient can be the result of a dense distribution of extracellular matrix structures or decreased water content, which allows greater absorption of light.32 Our previous study, which involved OCT evaluation of a facial skin laser treatment, demonstrated a decreased attenuation coefficient.34 This suggests that thermal heating with radiofrequency, as in the current study, induces a skin rejuvenation that differs from the physical damage done by lasers.
The OCT device has been utilized to review blood vessel quality and observe changes in blood flow related to potential inflammatory responses in dermatologic disease processes.32,34-37 In this study, an increase in blood flow was noted at several depths, particularly at 7 days posttreatment. This may be correlated to an early inflammatory response, consistent with the statistically significant increase in expression of inflammatory-related genes. However, blood flow decreased significantly at 3 and 6 months (Figure 13). Our recent studies with microneedling documented a similar pattern,37 whereas treatment of facial skin with lasers resulted in initial increase in blood flow and a return to basal levels at later time points similar to normal full-thickness wound healing.34,38
A limited number of studies have used biopsies to histologically evaluate skin diseases and aesthetic treatments. Furthermore, core microbiopsies with a 0.33-mm diameter skin punch have only been utilized in an aesthetic study of the skin following laser treatment, microneedling, and microbiopsy.34,36,37 This size of core microbiopsy is novel and has not been utilized in an aesthetic study related to the treatment of suprapatellar skin. These biopsies, which are virtually painless and greatly minimize the risk of scarring, can be processed to examine histologic, ultrastructural and gene expression analysis, providing a more objective assessment of cosmetic procedures.
Limitations of this study include a small population, comprising only females with a Fitzpatrick skin type between types I and III. Additionally, the follow-up visits for several subjects occurred during the summer months. Increased sun exposure during this time might have altered skin pigmentation and texture, which may have affected some noninvasive skin assessments and photographic evaluations.
Although the results of this study showed only the mildest of clinical improvements, any energy-based device could, in theory, improve skin laxity in the suprapatellar region. Anecdotally, our team has tried many different devices, but has been unable to achieve consistent, reproducible results. Future studies may explore the potential of other energy-based devices for treating skin laxity in this area.
CONCLUSIONS
Although radiofrequency devices have been shown to improve facial skin, the current study suggests that radiofrequency with microneedling to the suprapatellar skin has limited effects in decreasing skin laxity and improving skin appearance. At a molecular level, a single bipolar radiofrequency treatment of suprapatellar skin resulted in tissue rejuvenation. Further studies should evaluate multiple-treatment regimens with radiofrequency treatments along with combination treatment with lasers to safely induce profound skin rejuvenation.
Disclosures
Drs Kislevitz, Wamsley, Hoopman, and Kenkel report sponsor-supported funding from Venus Concept (Toronto, Ontario, Canada) and Bellus Medical (Addison, TX) for research studies outside of this submitted work. The other authors declared no potential conflicts of interest with respect to the research, authorship, and publication of this article.
Funding
This study was funded by the Department of Plastic Surgery, UT Southwestern Medical Center, Dallas, TX.
REFERENCES
Author notes
Editor-in-Chief of ASJ Open Forum; and Associate Editor of the Aesthetic Surgery Journal
- ultrasonography
- vascular flow
- gene expression
- epidermis
- collagen
- cutis laxa
- elastin
- genes
- hyaluronic acid
- diagnostic imaging
- histology
- interleukin-6
- skin
- interleukin-1 beta
- dermoepidermal junction
- optical coherence tomography
- transepidermal water loss rate
- radiofrequency therapy
- levels of evidence
- examination of skin
- skin elasticity
- attenuation