-
PDF
- Split View
-
Views
-
Cite
Cite
Michael Opoku Adomako, Peter Alpert, Dao-Lin Du, Fei-Hai Yu, Effects of fragmentation of clones compound over vegetative generations in the floating plant Pistia stratiotes, Annals of Botany, Volume 127, Issue 1, January 2021, Pages 123–133, https://doi.org/10.1093/aob/mcaa150
- Share Icon Share
Abstract
Clonal plants dominate many plant communities, especially in aquatic systems, and clonality appears to promote invasiveness and to affect how diversity changes in response to disturbance and resource availability. Understanding how the special physiological and morphological properties of clonal growth lead to these ecological effects depends upon studying the long-term consequences of clonal growth properties across vegetative generations, but this has rarely been done. This study aimed to show how a key clonal property, physiological integration between connected ramets within clones, affects the response of clones to disturbance and resources in an aquatic, invasive, dominant species across multiple generations.
Single, parental ramets of the floating stoloniferous plant Pistia stratiotes were grown for 3 weeks, during which they produced two or three generations of offspring; connections between new ramets were cut or left intact. Individual offspring were then used as parents in a second 3-week iteration that crossed fragmentation with previous fragmentation in the first iteration. A third iteration yielded eight treatment combinations, zero to three rounds of fragmentation at different times in the past. The experiment was run once at a high and once at a low level of nutrients.
In each iteration, fragmentation increased biomass of the parental ramet, decreased biomass of the offspring and increased number of offspring. These effects persisted and compounded from one iteration to another, though more recent fragmentation had stronger effects, and were stronger at the low than at the high nutrient level. Fragmentation did not affect net accumulation of mass by groups after one iteration but increased it after two iterations at low nutrients, and after three iterations at both nutrient levels.
Both the positive and negative effects of fragmentation on clonal performance can compound and persist over time and can be stronger when resource levels are lower. Even when fragmentation has no short-term net effect on clonal performance, it can have a longer-term effect. In some cases, fragmentation may increase total accumulation of mass by a clone. The results provide the first demonstration of how physiological integration in clonal plants can affect fitness across generations and suggest that increased disturbance may promote invasion of introduced clonal species via effects on integration, perhaps especially at lower nutrient levels.
INTRODUCTION
Clonal plants dominate many habitats, and a number of clonal species form extensive, nearly pure monocultures in wetlands (Aguilera et al., 2010; Travis et al., 2010; Zhang et al., 2010; Wang et al., 2020). Clonal growth is also associated with invasiveness in introduced plants (Pyšek et al., 1995; Liu et al., 2006; Wang et al., 2017; Nuñez-Mir et al., 2019), and decrease in plant diversity due to nutrient enrichment is due in part to an increase in the relative abundance of clonal species (Gough et al., 2012; Dickson and Gross, 2013; Dickson et al., 2014). Increased disturbance, which is linked to increase in the invasibility of plant communities (Alpert et al., 2000), can likewise increase the competitive ability of clonal plants (Wang et al., 2016). It thus seems likely that the special properties of clonal growth in plants may underlie important effects of changes in nutrient levels, disturbance and species introductions on natural communities and systems. However, linking clonal properties to their ecological consequences requires studies that span multiple vegetative generations, which remain rare (Dong et al., 2018, 2019). The aim of this study was to test the effects of physiological integration on the performance of a widespread invasive clonal plant subjected to contrasting levels of disturbance and nutrients over three generations.
The defining feature of clonal growth in plants is the production of vegetative offspring, or ramets, that retain a vascular connection to the parental plant at least until they become able to take up sufficient resources to survive on their own (de Kroon and van Groenendael, 1997; Münzbergová and Hadincová, 2017; Jaafry et al., 2018). Connections between ramets often persist long enough to form groups of several to many connected ramets (Alpert, 1990; Araki and Ohara, 2008; Moola and Vasseur, 2009). Fragmentation of these groups by disturbances that sever connections can strongly affect the individual performance of ramets in several ways.
First, when connected ramets are located in microsites with different levels of resource availability, fragmentation very often decreases growth of the ramets in microsites with relatively low resources (Alpert, 1999; Roiloa and Retuerto, 2012; Jaafry et al., 2018). In homogeneous environments, fragmentation can have similar effects if connected ramets are at different developmental stages such that they differ in capacity to take up resources (Roiloa et al., 2014; Dong et al., 2015). Second, fragmentation from the parental ramet can increase the production of new ramets by offspring (Oborny and Kun, 2001; Riis et al., 2010; Dong et al., 2012; Wang et al., 2016), possibly by a mechanism akin to release from apical dominance. Third, fragmentation can prevent signalling between ramets for other patterns of coordinated growth, such as division of labour (Alpert and Stuefer, 1997; Roiloa et al., 2007) and avoidance of below-ground competition (Holzapfel and Alpert, 2003; Gruntman and Novoplansky, 2004).
How these effects of fragmentation on individual ramets sum to affect their combined performance and thus clonal performance is less clear. Numerous studies have measured effects of initial fragmentation on total accumulation of dry mass and net production of new ramets by very small groups of two to several ramets (e.g. Song et al., 2013); these studies suggest that severing the connection between two ramets either decreases or has little effect on their combined growth. A few studies, all apparently on freshwater species, have further tested effects of continued fragmentation as new ramets are produced (Dong et al., 2012; Wang et al., 2016; Zhou et al., 2017a); these studies have found that severing connections between all ramets once they are able to survive increases or has little effect on their combined growth in the absence of competition. In the presence of competition, fragmentation of groups of ramets may decrease clonal growth (Wang et al., 2008, 2014; Yu et al., 2009), especially if nutrient levels are low. A third set of studies has compared the survival and growth of groups of one to several ramets produced by fragmentation of a larger group (Dong et al., 2012; Lin et al., 2012; Zhang et al., 2019) and generally found that larger groups have higher survival or growth.
No previous studies appear to have tested the longer-term effects of fragmentation on clonal performance across multiple vegetative generations. Recent publications on the transmission of transgenerational effects of temperature, herbivory and resource levels via vegetative reproduction in clones (Latzel et al., 2010; Gonzáles et al., 2016; Dong et al., 2018, 2019) suggest that effects of fragmentation on ramets might also persist between generations after disturbance. Continued disturbance could then compound effects of fragmentation, resulting in long-term effects not apparent in the short term (Burgess and Marshall, 2014).
We therefore asked three specific novel questions: (1) can effects of previous fragmentation persist after fragmentation has ceased? (2) can effects of fragmentation compound when previous fragmentation is repeated? and (3) is persistence or compounding of fragmentation effects greater when resource levels are lower? We addressed these questions in a greenhouse experiment on the widespread, floating, stoloniferous, invasive species Pistia stratiotes. Clonal plants that float on lakes and rivers are probably especially vulnerable to fragmentation, both because disturbance is often frequent and because connections between ramets tend to be non-lignified and relatively fragile (Room, 1983; Barrat-Segretain, 1996; Barrat-Segretain and Bornette, 2000). Some floating clonal species, including P. stratiotes, are also of particular ecological interest because they can become very abundant and be highly invasive following introduction, largely or entirely due to clonal growth (Holm et al., 1997; Zhang et al., 2010).
MATERIALSAND METHODS
Species and propagation
Pistia stratiotes, or water lettuce, is a perennial, floating, aquatic macrophyte in the Araceae that occurs in tropical to subtropical regions around the world (Evans, 2013; Hussner et al., 2014). Individual ramets consist of a short, unbranched stem with a rosette of broadly spathulate leaves up to 20 cm long and numerous roots. Ramets initiate stolons proximal to the leaves; stolons grow up to ~20 cm long and end in a new ramet. Plants can produce a new vegetative generation in <2 weeks under favourable conditions, and P. stratiotes often forms extensive mats on the surface of slow-moving water. Pistia stratiotes is considered invasive in China and other countries because mats can reduce light and oxygen levels in the water beneath and interfere with human activities (Howard and Harley, 1998; Zhou et al., 2017b)
Plants were collected on 17 May 2018 in Wenzhou (27°59′39″ N, 120°41′57″ E), Zhejiang Province, in southern China, transported ~120 km north to a greenhouse at the Jiaojiang Campus of Taizhou University in Taizhou, Zhejiang Province, and propagated in a tank filled with tap water. On 1 June 2018, 120 ramets of approximately equal size were selected and moved to a tank containing a nutrient solution of 4.5 mg water-soluble fertilizer per litre (Peters Professional 20-20-20 General Purpose Fertilizer; Everris, NA, USA: 20 % total N, 20 % available PO4, 20 % soluble potash, 0.05 % Mg, 0.05 % Fe, 0.025 % Mn, 0.025 % Zn, 0.0125 % B, 0.0125 % Cu and 0.005 % Mo). After 2 months, during which the nutrient solution was replaced about every 2 weeks, 72 of the second-oldest offspring of the original ramets were detached for use in the experiment and moved to a separate tank filled with nutrient solution for 14 d. One objective of this protocol was to reduce any effects of prior exposure to varying environmental conditions in the field.
Overview of the experimental design
To test effects of repeated fragmentation at different levels of nutrient availability on the performance of ramets of P. stratiotes, the experiment was run twice, once at a high level of nutrients and once at a low level. The levels were chosen to lie within the range of those likely to be found in wetlands where the species occurs in China and to differ enough to affect growth. The large size of the experiment and the limited resources available precluded running nutrient levels simultaneously, but they overlapped in time as much as possible. Each run consisted of three iterations of two fragmentation treatments, fragmented and not fragmented; for details see the section of Treatments and measurements (below).
Each iteration started from single ramets taken from the first vegetative generation in the previous iteration and lasted 3 weeks (Fig. 1). Treatments compounded from iteration to iteration. The first iteration had just two treatment combinations, fragmented and not fragmented. The second iteration had four treatment combinations, fragmented and not fragmented in the second iteration crossed with fragmented and not fragmented in the first iteration. The third iteration had eight treatment combinations, fragments and not fragmented in the third iteration crossed with the four treatment combinations in the second iteration. The third iteration thus had one treatment combination in which plants had not been fragmented in any iteration, three combinations in which plants had been fragmented in one iteration (first, second or third), three combinations in which plants had been fragmented in two iterations (first and second, first and third, or second and third), and one combination in which plants had been fragmented in all three iterations.
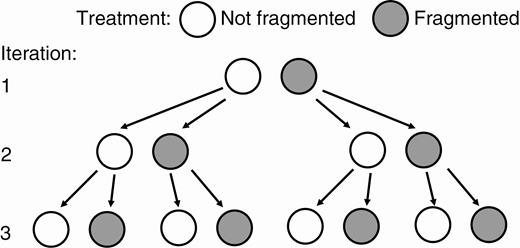
Experimental design. The first iteration began with single ramets whose vegetative offspring were either severed (fragmented treatment) or left connected (not fragmented). The second iteration began with single offspring of the original ramets in the first iteration, and the third iteration with offspring from the second iteration. The experiment was run once at a high level of nutrients and once at a low level. See text for details.
Twelve replicates of each treatment combination in each iteration were harvested at the end of the iteration and measured for size and mass. Within an iteration, the starting ramet is termed the primary ramet, its offspring are termed secondary ramets, and their offspring are termed tertiary ramets. Since each iteration started with the offspring of the starting ramet in the previous iteration, secondary ramets in one iteration became the primary ramets in the next iteration. It would have been ideal to follow the fates of all ramets in the clonal fragments, but this would have required an experiment of unmanageable size. Instead, growth was followed in the first-generation offspring produced by initial, single ramets such as might be dispersed into a new habitat.
Treatments and measurements
On 15 August 2018, 12 of the 72 ramets prepared for the experiment were randomly selected for measurements of initial size. After removal of any new stolons, these ramets were measured for size, separated into roots and leaves plus stem, oven-dried at 70 °C for 48 h and weighed. Initial dry mass (mean ± s.e.) was: roots 0.04 ± 0.02 g; leaves plus stem 0.39 ± 0.05 g; total 0.42 ± 0.07 g. Ramets had five or six leaves, a mean longest root length of 26 cm and a mean rosette diameter of 14.3 cm.
The remaining 60 ramets were each placed in a 21-L plastic bucket 32 cm tall by 36 cm in diameter filled with 15 L of 20 % Hoagland solution. Thirty ramets were then randomly assigned to each of the two fragmentation treatments, fragmented and not fragmented. In the fragmented treatment, offspring (secondary ramets) of the starting ramet (primary ramet) and the offspring of the offspring (tertiary ramets) were detached by cutting the proximal stolon. All ramets were thus severed from each other, except that no attempt was made to detach any offspring produced by tertiary ramets because these offspring remained very small. Cutting was done every 5 d. The nutrient solution was stirred and skimmed with a net daily to remove any algae; the solution was replaced weekly.
After 21 d, 12 randomly selected replicates of each fragmentation treatment were washed with deionized water to remove nutrient solution and blotted dry with tissue paper. The secondary and tertiary ramets were counted. Each replicate was separated into the primary ramet, the secondary ramets and the tertiary ramets plus any new stolons and ramets they had produced, dried at 70 °C for 48 h, and weighed.
Twelve of the remaining replicates of each fragmentation treatment from the first iteration were randomly selected for the second iteration of treatments. The remaining six replicates were discarded. Four secondary ramets were randomly selected in each of the 12 replicates of each fragmentation treatment, and randomly assigned to one of the two fragmentation treatments, fragmented or not fragmented, with two ramets each. Thus, there were 24 replicates in each of the four treatment combinations in the second iteration, fragmented or not in the first iteration crossed with fragmented or not in the second iteration. After 3 weeks, half of the replicates in each treatment combination were harvested as described and the other half were used for the third and final iteration of treatments. The third iteration followed the same procedure as the second except that only two secondary ramets from each of the 12 replicates that were not harvested in the second iteration were assigned to each of the two fragmentation treatments in the third iteration. Therefore, there were eight treatments in the third iteration, i.e. fragmented or not in the first iteration crossed with fragmented or not in the second iteration crossed with fragmented or not in the third iteration, and each had 12 replicates. All the replicates of the third iteration were harvested after 3 more weeks, on 16 October 2018.
To test how low nutrient availability might change the effects of fragmentation, the experiment was also run using 5 % Hoagland solution. Treatments for the low-nutrient run began when the third iteration of the high-nutrient run started, on 26 September, and ended on 27 November 2018. Over the entire experimental period, mean temperature in the greenhouse was 24.8 ± 0.07 °C and humidity was 87.2 ± 0.25 %, both based on hourly measurements.
Data analysis
For the first iteration of each run, one-way ANOVAs were used to test the effect of fragmentation (fragmented or not, fixed effect) on mass of the primary ramet; total mass, mass per ramet and number of secondary ramets; total mass, mass per ramet and number of tertiary ramets; and final total dry mass of all ramets together. For the second iteration, two-way ANOVAs were used to test effects of fragmentation during the first iteration and fragmentation during the second iteration on the same set of dependent variables. For the third iteration, three-way ANOVAs were used to test effects of fragmentation in the first, in the second and in the third iteration.
Data were transformed as needed to reduce heteroscedasticity. Transformed data for the high-nutrient run were as follows: (first iteration) mass per tertiary ramet and number of tertiary ramets (log); (second iteration) mass per primary ramet, mass per secondary ramet, mass per tertiary ramet and number of tertiary ramets (log), and mass of secondary ramets (square root); (third iteration) all variables (log). Transformed data for the low-nutrient run were as follows: (first iteration) number of secondary ramets, mass of secondary ramets, mass per secondary ramet and number of tertiary ramets (log), and mass per primary ramet, mass of tertiary ramets and mass per tertiary ramet (square root); (second iteration) mass of secondary ramets (square root) and other dependent variables except number and mass of tertiary ramets (log); (third iteration) all variables (log). Figures show untransformed data. To facilitate comparison between vegetative generations, which differed by up to two magnitudes in mass per ramet, the vertical axes of graphs are marked using the same minimum units where possible, such as using ticks every 0.2 g, but with the spacing between ticks varied as needed. Analyses were performed using SPSS (v20.0; IBM Corp., Armonk, NY, USA).
RESULTS
First iteration
In the first iteration with high nutrients, fragmentation increased final dry mass of the starting, or primary, ramet (Fig. 2B) and decreased mass of its offspring combined (mass of secondary ramets, Fig. 2C) and of their offspring combined (mass of tertiary ramets; Fig. 2F). Mass per ramet in the secondary and tertiary ramets decreased by 40–60 % (Fig. 2D, G). The number of secondary ramets did not change significantly (P > 0.1, Fig. 2E), but the number of tertiary ramets increased by ~60 % (Fig. 2H). Because mass of the primary ramet was over 10 times greater than mass per secondary ramet and over 40 times greater than mass per tertiary ramet, the net result of the positive effect of fragmentation on mass of the primary ramet and the negative effect on mass of the secondary and tertiary ramets was that fragmentation had little effect on total mass of all ramets combined (Fig. 2A).
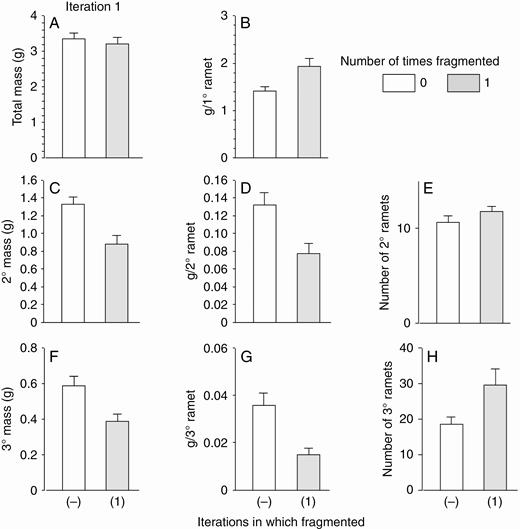
High-nutrient run: effects of the first iteration of fragmentation treatments on final total dry mass (mean + s.e.) of clonal fragments and mass, mass per ramet, and number of ramets in the parental (primary) ramet (1°), its offspring (secondary ramets, 2°) and their offspring (tertiary ramets, 3°). There was only one parental ramet, and thus mass per primary ramet equals mass of the primary ramet. ANOVA (effect of fragmentation, d.f. 1,22): total mass, F = 0.3, P = 0.6; mass per primary ramet, F = 7.9, P = 0.01; mass of secondary ramets, F = 12.5, P = 0.002; mass per secondary ramet, F = 9.7, P = 0.005; number of secondary ramets, F = 1.9, P = 0.2; mass of tertiary ramets, F = 9.3, P = 0.006; mass per tertiary ramet, F = 20.8, P < 0.001; number of tertiary ramets, F = 4.8, P = 0.04.
With low nutrients, most effects of the first iteration of fragmentation were qualitatively similar but stronger (Fig. 3). Final mass of the primary ramet was four times greater in the fragmented than in the not-fragmented treatment (Fig. 3B), mass per secondary and tertiary ramet was 85–90 % less (Fig. 3C), and the number of tertiary ramets was more than two times greater (Fig. 3F). One effect of fragmentation was seen with low but not with high nutrients: the number of secondary ramets was two times greater in the fragmented than in the not-fragmented treatment (Fig. 3E). Across fragmentation treatments in the first iteration of each run, total mass of all ramets combined (Fig. 3A) was only about one-third as large with low nutrients as with high nutrients (Fig. 2A).
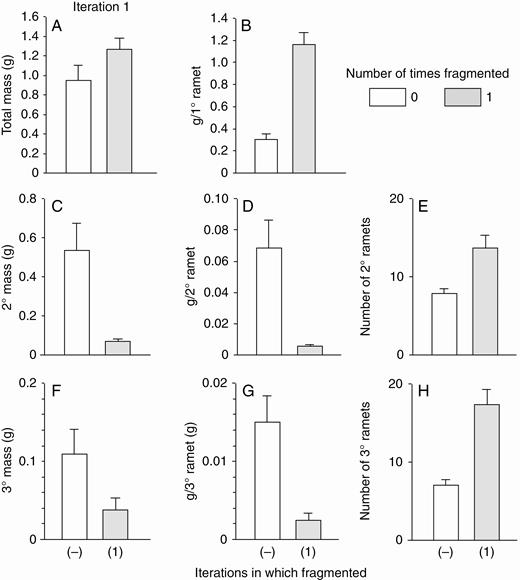
Low-nutrient run: effects of the first iteration of fragmentation treatments on final total dry mass (mean + s.e.) of clonal fragments and mass, mass per ramet, and number of ramets in the parental (primary) ramet (1°), its offspring (secondary ramets, 2°) and their offspring (tertiary ramets, 3°). There was only one parental ramet, and thus mass per primary ramet equals mass of the primary mass ramet. ANOVA (effect of fragmentation, d.f. 1,22): total mass, F = 2.7, P = 0.11; mass per primary ramet, F = 55.9, P < 0.001; mass of secondary ramets, F = 9.7, P = 0.005; mass per secondary ramet, F = 18.9, P < 0.001; number of secondary ramets, F = 12.7, P = 0.002; mass of tertiary ramets, F = 9.2, P = 0.006; mass per tertiary ramet, F = 31.2, P < 0.001; number of tertiary ramets, F = 24.7, P < 0.001.
Second iteration
With high nutrients, fragmentation in the second iteration again increased mass of the primary ramet (Fig. 4B, first two versus second two bars; Table 1, effect of fragmentation in second iteration) and decreased the combined and individual mass of secondary (Fig. 4C, D; Table 1) and tertiary ramets (Fig. 4F, G; Table 1). Fragmentation in the second iteration with high nutrients again had no effect on total mass of all ramets combined (Fig. 4A; Table 1) but increased the number of both secondary (Fig. 4E) and tertiary ramets (Fig. 4F; Table 1).
ANOVAs for the second iterations of the runs with high and low nutrients. d.f. = 1,44. Values are in bold where P < 0.01 and in italics where P = 0.01–0.05
Variable . | Fragmentation in first iteration (F1) . | Fragmentation in second iteration (F2) . | F1 × F2 . | |||
---|---|---|---|---|---|---|
F | P | F | P | F | P | |
High nutrients | ||||||
Total mass | 0.9 | 0.4 | 0.5 | 0.5 | 0.7 | 0.4 |
Mass per primary ramet | 0.3 | 0.6 | 15.0 | <0.001 | 0.2 | 0.7 |
Mass of secondary ramets | 19.4 | <0.001 | 25.7 | <0.001 | <0.1 | >0.9 |
Mass per secondary ramet | 6.7 | 0.013 | 46.0 | <0.001 | 0.3 | 0.6 |
No. of secondary ramets | 1.2 | 0.3 | 16.5 | <0.001 | 0.3 | 0.6 |
Mass of tertiary ramets | 4.8 | 0.03 | 4.7 | 0.04 | <0.1 | >0.9 |
Mass per tertiary ramet | 0.1 | 0.8 | 20.4 | <0.001 | 4.4 | 0.04 |
No. of tertiary ramets | 3.0 | 0.09 | 15.0 | <0.001 | 1.1 | 0.3 |
Low nutrients | ||||||
Total mass | 47.0 | <0.001 | 62.3 | <0.001 | 23.5 | <0.001 |
Mass per primary ramet | 77.3 | <0.001 | 151.4 | <0.001 | 6.5 | 0.02 |
Mass of secondary ramets | 2.9 | 0.09 | 10.8 | 0.002 | 1.1 | 0.3 |
Mass per secondary ramet | 12.1 | 0.001 | 22.5 | <0.001 | 0.7 | 0.4 |
No. of secondary ramets | 9.7 | 0.003 | 10.6 | 0.002 | <0.1 | >0.9 |
Tertiary mass Mass of tertiary ramets | 0.5 | 0.5 | 8.5 | 0.006 | <0.1 | >0.9 |
Mass per tertiary ramet | 5.8 | 0.02 | 47.9 | <0.001 | 0.2 | 0.7 |
No. of tertiary ramets | 5.6 | 0.02 | 30.2 | <0.001 | 1.6 | 0.2 |
Variable . | Fragmentation in first iteration (F1) . | Fragmentation in second iteration (F2) . | F1 × F2 . | |||
---|---|---|---|---|---|---|
F | P | F | P | F | P | |
High nutrients | ||||||
Total mass | 0.9 | 0.4 | 0.5 | 0.5 | 0.7 | 0.4 |
Mass per primary ramet | 0.3 | 0.6 | 15.0 | <0.001 | 0.2 | 0.7 |
Mass of secondary ramets | 19.4 | <0.001 | 25.7 | <0.001 | <0.1 | >0.9 |
Mass per secondary ramet | 6.7 | 0.013 | 46.0 | <0.001 | 0.3 | 0.6 |
No. of secondary ramets | 1.2 | 0.3 | 16.5 | <0.001 | 0.3 | 0.6 |
Mass of tertiary ramets | 4.8 | 0.03 | 4.7 | 0.04 | <0.1 | >0.9 |
Mass per tertiary ramet | 0.1 | 0.8 | 20.4 | <0.001 | 4.4 | 0.04 |
No. of tertiary ramets | 3.0 | 0.09 | 15.0 | <0.001 | 1.1 | 0.3 |
Low nutrients | ||||||
Total mass | 47.0 | <0.001 | 62.3 | <0.001 | 23.5 | <0.001 |
Mass per primary ramet | 77.3 | <0.001 | 151.4 | <0.001 | 6.5 | 0.02 |
Mass of secondary ramets | 2.9 | 0.09 | 10.8 | 0.002 | 1.1 | 0.3 |
Mass per secondary ramet | 12.1 | 0.001 | 22.5 | <0.001 | 0.7 | 0.4 |
No. of secondary ramets | 9.7 | 0.003 | 10.6 | 0.002 | <0.1 | >0.9 |
Tertiary mass Mass of tertiary ramets | 0.5 | 0.5 | 8.5 | 0.006 | <0.1 | >0.9 |
Mass per tertiary ramet | 5.8 | 0.02 | 47.9 | <0.001 | 0.2 | 0.7 |
No. of tertiary ramets | 5.6 | 0.02 | 30.2 | <0.001 | 1.6 | 0.2 |
ANOVAs for the second iterations of the runs with high and low nutrients. d.f. = 1,44. Values are in bold where P < 0.01 and in italics where P = 0.01–0.05
Variable . | Fragmentation in first iteration (F1) . | Fragmentation in second iteration (F2) . | F1 × F2 . | |||
---|---|---|---|---|---|---|
F | P | F | P | F | P | |
High nutrients | ||||||
Total mass | 0.9 | 0.4 | 0.5 | 0.5 | 0.7 | 0.4 |
Mass per primary ramet | 0.3 | 0.6 | 15.0 | <0.001 | 0.2 | 0.7 |
Mass of secondary ramets | 19.4 | <0.001 | 25.7 | <0.001 | <0.1 | >0.9 |
Mass per secondary ramet | 6.7 | 0.013 | 46.0 | <0.001 | 0.3 | 0.6 |
No. of secondary ramets | 1.2 | 0.3 | 16.5 | <0.001 | 0.3 | 0.6 |
Mass of tertiary ramets | 4.8 | 0.03 | 4.7 | 0.04 | <0.1 | >0.9 |
Mass per tertiary ramet | 0.1 | 0.8 | 20.4 | <0.001 | 4.4 | 0.04 |
No. of tertiary ramets | 3.0 | 0.09 | 15.0 | <0.001 | 1.1 | 0.3 |
Low nutrients | ||||||
Total mass | 47.0 | <0.001 | 62.3 | <0.001 | 23.5 | <0.001 |
Mass per primary ramet | 77.3 | <0.001 | 151.4 | <0.001 | 6.5 | 0.02 |
Mass of secondary ramets | 2.9 | 0.09 | 10.8 | 0.002 | 1.1 | 0.3 |
Mass per secondary ramet | 12.1 | 0.001 | 22.5 | <0.001 | 0.7 | 0.4 |
No. of secondary ramets | 9.7 | 0.003 | 10.6 | 0.002 | <0.1 | >0.9 |
Tertiary mass Mass of tertiary ramets | 0.5 | 0.5 | 8.5 | 0.006 | <0.1 | >0.9 |
Mass per tertiary ramet | 5.8 | 0.02 | 47.9 | <0.001 | 0.2 | 0.7 |
No. of tertiary ramets | 5.6 | 0.02 | 30.2 | <0.001 | 1.6 | 0.2 |
Variable . | Fragmentation in first iteration (F1) . | Fragmentation in second iteration (F2) . | F1 × F2 . | |||
---|---|---|---|---|---|---|
F | P | F | P | F | P | |
High nutrients | ||||||
Total mass | 0.9 | 0.4 | 0.5 | 0.5 | 0.7 | 0.4 |
Mass per primary ramet | 0.3 | 0.6 | 15.0 | <0.001 | 0.2 | 0.7 |
Mass of secondary ramets | 19.4 | <0.001 | 25.7 | <0.001 | <0.1 | >0.9 |
Mass per secondary ramet | 6.7 | 0.013 | 46.0 | <0.001 | 0.3 | 0.6 |
No. of secondary ramets | 1.2 | 0.3 | 16.5 | <0.001 | 0.3 | 0.6 |
Mass of tertiary ramets | 4.8 | 0.03 | 4.7 | 0.04 | <0.1 | >0.9 |
Mass per tertiary ramet | 0.1 | 0.8 | 20.4 | <0.001 | 4.4 | 0.04 |
No. of tertiary ramets | 3.0 | 0.09 | 15.0 | <0.001 | 1.1 | 0.3 |
Low nutrients | ||||||
Total mass | 47.0 | <0.001 | 62.3 | <0.001 | 23.5 | <0.001 |
Mass per primary ramet | 77.3 | <0.001 | 151.4 | <0.001 | 6.5 | 0.02 |
Mass of secondary ramets | 2.9 | 0.09 | 10.8 | 0.002 | 1.1 | 0.3 |
Mass per secondary ramet | 12.1 | 0.001 | 22.5 | <0.001 | 0.7 | 0.4 |
No. of secondary ramets | 9.7 | 0.003 | 10.6 | 0.002 | <0.1 | >0.9 |
Tertiary mass Mass of tertiary ramets | 0.5 | 0.5 | 8.5 | 0.006 | <0.1 | >0.9 |
Mass per tertiary ramet | 5.8 | 0.02 | 47.9 | <0.001 | 0.2 | 0.7 |
No. of tertiary ramets | 5.6 | 0.02 | 30.2 | <0.001 | 1.6 | 0.2 |
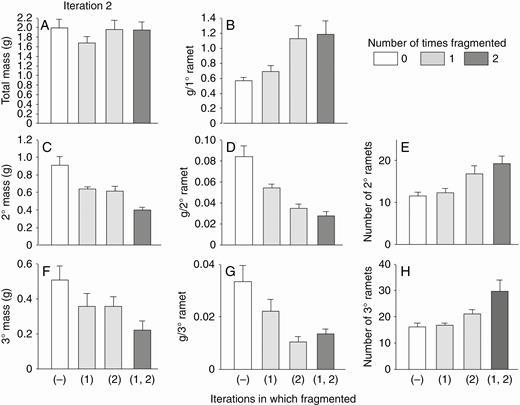
High-nutrient run: effects of the second iteration of fragmentation treatments on final total dry mass (mean + s.e.) of clonal fragments and mass, mass per ramet, and number of ramets in the parental (primary) ramet (1°), its offspring (secondary ramets, 2°) and their offspring (tertiary ramets, 3°). There was only one parental ramet, and thus mass per primary ramet equals mass of the primary ramets. The parental ramet was a secondary ramet in the first iteration.
With high nutrients, fragmentation in the first iteration did not affect final mass of primary ramets in the second iteration (Fig. 4B; Table 1: effect of fragmentation in first iteration). However, primary ramets from plants fragmented in the first iteration did produce less total mass of secondary ramets and tertiary ramets than primary ramets not fragmented in the first iteration (Fig. 4C, F; Table 1). There was little significant interaction between the effects of fragmentation in the first and second iterations (Table 1: effect of F1 × F2). Ending masses were generally less than in the first iteration (Fig. 2) but numbers of new ramets were not.
With low nutrients, effects of the second iteration of fragmentation were again mostly qualitatively similar to effects with high nutrients but stronger (Fig. 5; Table 1). One main difference from results with high nutrients was that fragmentation with low nutrients greatly increased mass of all ramets combined (Fig. 5A; Table 1). This was largely because mass of the primary ramet was more than twice as high in the fragmented as in the not-fragmented treatment (Fig. 5B). Another difference was that previous fragmentation in the first iteration increased mass of all ramets combined, again largely because of positive effects on the primary ramet. Effects of fragmentation in the first and second iterations interacted (Table 1, effect of F1 × F2): mass of the primary ramet and of all ramets combined was three to four times higher if plants had been fragmented in both iterations than if they had not been fragmented in one or both iterations (Fig. 5A, B).
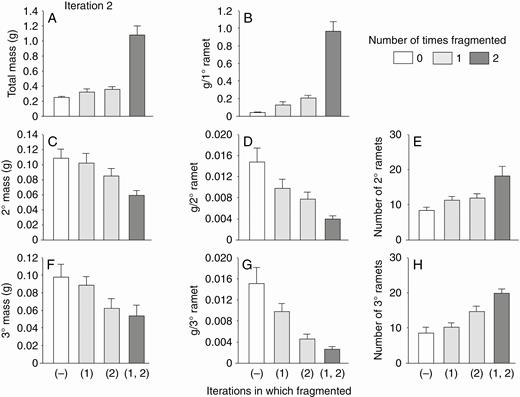
Low-nutrient run: effects of the second iteration of fragmentation treatments on final total dry mass (mean + s.e.) of clonal fragments and mass, mass per ramet, and number of ramets in the parental (primary) ramet (1°), its offspring (secondary ramets, 2°) and their offspring (tertiary ramets, 3°). There was only one parental ramet, and thus mass per primary ramet equals mass of the primary ramets. The parental ramet was a secondary ramet in the first iteration.
Third iteration
The third iteration with high nutrients continued to show strong positive effects of fragmentation on mass per primary ramet and numbers of secondary and tertiary ramets, and strong negative effects of fragmentation on mass per secondary and tertiary ramet (Fig. 6; Table 2). Plants also showed numerous strong, parallel effects of fragmentation in previous iterations. Effects were generally larger when the number of fragmentation treatments was greater, with the largest effects in plants fragmented in all three iterations [Fig. 6, treatment marked ‘(1, 2, 3)’]. There was also a tendency for effects of more recent fragmentations to be greater. For example, among plants fragmented in two iterations, effects were stronger in those fragmented in the second and third iterations than in those fragmented in the first and second or in the first and third iterations.
ANOVAs for the third iterations of the runs with high and low nutrients. d.f. = 1,88. Values are in bold where P < 0.01 and in italics where P = 0.01–0.05
Variable . | Fragmentation in first iteration (F1) . | Fragmentation in second iteration (F2) . | Fragmentation in third iteration (F3) . | F1 × F2 . | F1 × F3 . | F2 × F3 . | F1 × F2 × F3 . | |||||||
---|---|---|---|---|---|---|---|---|---|---|---|---|---|---|
F | P | F | P | F | P | F | P | F | P | F | P | F | P | |
High nutrient | ||||||||||||||
Total mass | 7.9 | 0.006 | 2.7 | 0.10 | 3.1 | 0.08 | <0.1 | 0.8 | 4.0 | 0.05 | 7.0 | 0.01 | 2.2 | 0.14 |
Mass per primary ramet | 22.9 | <0.001 | 20.9 | <0.001 | 42.1 | <0.001 | 0.5 | 0.5 | 0.7 | 0.4 | 1.6 | 0.2 | 4.9 | 0.03 |
Mass of secondary ramets | 1.0 | 0.3 | 12.7 | 0.001 | 25.9 | <0.001 | 0.9 | 0.3 | <0.1 | >0.9 | 0.4 | 0.5 | 0.2 | 0.7 |
Mass per secondary ramet | 5.5 | 0.02 | 28.0 | <0.001 | 43.8 | <0.001 | 0.3 | 0.6 | <0.1 | >0.9 | <0.1 | 0.9 | 0.4 | 0.5 |
No. of secondary ramets | 7.1 | 0.009 | 18.5 | <0.001 | 20.7 | <0.001 | 0.1 | 0.8 | <0.1 | >0.9 | 0.3 | 0.6 | 2.6 | 0.11 |
Mass of tertiary ramets | 2.7 | 0.10 | 23.6 | <0.001 | 41.2 | <0.001 | <0.1 | >0.9 | <0.1 | 0.8 | 0.3 | 0.6 | 0.1 | 0.8 |
Mass per tertiary ramet | 8.1 | 0.005 | 36.8 | <0.001 | 70.4 | <0.001 | 0.4 | 0.6 | 0.3 | 0.6 | <0.1 | >0.9 | 0.8 | 0.4 |
No. of tertiary ramets | 5.6 | 0.02 | 12.2 | 0.001 | 26.8 | <0.001 | 0.7 | 0.4 | 1.0 | 0.3 | 0.2 | 0.6 | 1.0 | 3.0 |
Low nutrients | ||||||||||||||
Total mass | 3.6 | 0.06 | 0.5 | 0.5 | 4.9 | 0.03 | 0.9 | 0.3 | 8.0 | 0.006 | 26.1 | <0.001 | 1.8 | 0.18 |
Mass per primary ramet | 17.5 | <0.001 | 28.5 | <0.001 | 48.2 | <0.001 | 0.3 | 0.6 | 9.4 | 0.003 | 10.5 | 0.002 | 0.9 | 0.3 |
Mass of secondary ramets | 4.2 | 0.04 | 23.8 | 0.001 | 26.8 | <0.001 | <0.1 | 0.9 | <0.1 | >0.9 | <0.1 | 0.9 | 2.6 | 0.11 |
Mass per secondary ramet | 10.1 | 0.002 | 34.9 | <0.001 | 49.9 | <0.001 | 0.7 | 0.4 | 0.7 | 0.4 | 0.6 | 0.4 | 1.8 | 0.2 |
No. of secondary ramets | 4.5 | 0.04 | 7.8 | 0.006 | 16.4 | <0.001 | 1.7 | 0.2 | 1.0 | 0.3 | 0.8 | 0.4 | <0.1 | >0.9 |
Mass of tertiary ramets | 9.0 | 0.003 | 29.6 | <0.001 | 22.8 | <0.001 | <0.1 | 0.8 | 0.3 | 0.6 | 2.9 | 0.09 | 12.9 | 0.001 |
Mass per tertiary ramet | 15.9 | <0.001 | 58.7 | <0.001 | 65.5 | <0.001 | 0.5 | 0.5 | 1.0 | 0.3 | 0.4 | 0.5 | 9.5 | 0.003 |
No. of tertiary ramets | 2.3 | 0.14 | 10.5 | 0.002 | 19.9 | <0.001 | 0.3 | 0.6 | 0.3 | 0.6 | 1.2 | 0.3 | <0.1 | 0.9 |
Variable . | Fragmentation in first iteration (F1) . | Fragmentation in second iteration (F2) . | Fragmentation in third iteration (F3) . | F1 × F2 . | F1 × F3 . | F2 × F3 . | F1 × F2 × F3 . | |||||||
---|---|---|---|---|---|---|---|---|---|---|---|---|---|---|
F | P | F | P | F | P | F | P | F | P | F | P | F | P | |
High nutrient | ||||||||||||||
Total mass | 7.9 | 0.006 | 2.7 | 0.10 | 3.1 | 0.08 | <0.1 | 0.8 | 4.0 | 0.05 | 7.0 | 0.01 | 2.2 | 0.14 |
Mass per primary ramet | 22.9 | <0.001 | 20.9 | <0.001 | 42.1 | <0.001 | 0.5 | 0.5 | 0.7 | 0.4 | 1.6 | 0.2 | 4.9 | 0.03 |
Mass of secondary ramets | 1.0 | 0.3 | 12.7 | 0.001 | 25.9 | <0.001 | 0.9 | 0.3 | <0.1 | >0.9 | 0.4 | 0.5 | 0.2 | 0.7 |
Mass per secondary ramet | 5.5 | 0.02 | 28.0 | <0.001 | 43.8 | <0.001 | 0.3 | 0.6 | <0.1 | >0.9 | <0.1 | 0.9 | 0.4 | 0.5 |
No. of secondary ramets | 7.1 | 0.009 | 18.5 | <0.001 | 20.7 | <0.001 | 0.1 | 0.8 | <0.1 | >0.9 | 0.3 | 0.6 | 2.6 | 0.11 |
Mass of tertiary ramets | 2.7 | 0.10 | 23.6 | <0.001 | 41.2 | <0.001 | <0.1 | >0.9 | <0.1 | 0.8 | 0.3 | 0.6 | 0.1 | 0.8 |
Mass per tertiary ramet | 8.1 | 0.005 | 36.8 | <0.001 | 70.4 | <0.001 | 0.4 | 0.6 | 0.3 | 0.6 | <0.1 | >0.9 | 0.8 | 0.4 |
No. of tertiary ramets | 5.6 | 0.02 | 12.2 | 0.001 | 26.8 | <0.001 | 0.7 | 0.4 | 1.0 | 0.3 | 0.2 | 0.6 | 1.0 | 3.0 |
Low nutrients | ||||||||||||||
Total mass | 3.6 | 0.06 | 0.5 | 0.5 | 4.9 | 0.03 | 0.9 | 0.3 | 8.0 | 0.006 | 26.1 | <0.001 | 1.8 | 0.18 |
Mass per primary ramet | 17.5 | <0.001 | 28.5 | <0.001 | 48.2 | <0.001 | 0.3 | 0.6 | 9.4 | 0.003 | 10.5 | 0.002 | 0.9 | 0.3 |
Mass of secondary ramets | 4.2 | 0.04 | 23.8 | 0.001 | 26.8 | <0.001 | <0.1 | 0.9 | <0.1 | >0.9 | <0.1 | 0.9 | 2.6 | 0.11 |
Mass per secondary ramet | 10.1 | 0.002 | 34.9 | <0.001 | 49.9 | <0.001 | 0.7 | 0.4 | 0.7 | 0.4 | 0.6 | 0.4 | 1.8 | 0.2 |
No. of secondary ramets | 4.5 | 0.04 | 7.8 | 0.006 | 16.4 | <0.001 | 1.7 | 0.2 | 1.0 | 0.3 | 0.8 | 0.4 | <0.1 | >0.9 |
Mass of tertiary ramets | 9.0 | 0.003 | 29.6 | <0.001 | 22.8 | <0.001 | <0.1 | 0.8 | 0.3 | 0.6 | 2.9 | 0.09 | 12.9 | 0.001 |
Mass per tertiary ramet | 15.9 | <0.001 | 58.7 | <0.001 | 65.5 | <0.001 | 0.5 | 0.5 | 1.0 | 0.3 | 0.4 | 0.5 | 9.5 | 0.003 |
No. of tertiary ramets | 2.3 | 0.14 | 10.5 | 0.002 | 19.9 | <0.001 | 0.3 | 0.6 | 0.3 | 0.6 | 1.2 | 0.3 | <0.1 | 0.9 |
ANOVAs for the third iterations of the runs with high and low nutrients. d.f. = 1,88. Values are in bold where P < 0.01 and in italics where P = 0.01–0.05
Variable . | Fragmentation in first iteration (F1) . | Fragmentation in second iteration (F2) . | Fragmentation in third iteration (F3) . | F1 × F2 . | F1 × F3 . | F2 × F3 . | F1 × F2 × F3 . | |||||||
---|---|---|---|---|---|---|---|---|---|---|---|---|---|---|
F | P | F | P | F | P | F | P | F | P | F | P | F | P | |
High nutrient | ||||||||||||||
Total mass | 7.9 | 0.006 | 2.7 | 0.10 | 3.1 | 0.08 | <0.1 | 0.8 | 4.0 | 0.05 | 7.0 | 0.01 | 2.2 | 0.14 |
Mass per primary ramet | 22.9 | <0.001 | 20.9 | <0.001 | 42.1 | <0.001 | 0.5 | 0.5 | 0.7 | 0.4 | 1.6 | 0.2 | 4.9 | 0.03 |
Mass of secondary ramets | 1.0 | 0.3 | 12.7 | 0.001 | 25.9 | <0.001 | 0.9 | 0.3 | <0.1 | >0.9 | 0.4 | 0.5 | 0.2 | 0.7 |
Mass per secondary ramet | 5.5 | 0.02 | 28.0 | <0.001 | 43.8 | <0.001 | 0.3 | 0.6 | <0.1 | >0.9 | <0.1 | 0.9 | 0.4 | 0.5 |
No. of secondary ramets | 7.1 | 0.009 | 18.5 | <0.001 | 20.7 | <0.001 | 0.1 | 0.8 | <0.1 | >0.9 | 0.3 | 0.6 | 2.6 | 0.11 |
Mass of tertiary ramets | 2.7 | 0.10 | 23.6 | <0.001 | 41.2 | <0.001 | <0.1 | >0.9 | <0.1 | 0.8 | 0.3 | 0.6 | 0.1 | 0.8 |
Mass per tertiary ramet | 8.1 | 0.005 | 36.8 | <0.001 | 70.4 | <0.001 | 0.4 | 0.6 | 0.3 | 0.6 | <0.1 | >0.9 | 0.8 | 0.4 |
No. of tertiary ramets | 5.6 | 0.02 | 12.2 | 0.001 | 26.8 | <0.001 | 0.7 | 0.4 | 1.0 | 0.3 | 0.2 | 0.6 | 1.0 | 3.0 |
Low nutrients | ||||||||||||||
Total mass | 3.6 | 0.06 | 0.5 | 0.5 | 4.9 | 0.03 | 0.9 | 0.3 | 8.0 | 0.006 | 26.1 | <0.001 | 1.8 | 0.18 |
Mass per primary ramet | 17.5 | <0.001 | 28.5 | <0.001 | 48.2 | <0.001 | 0.3 | 0.6 | 9.4 | 0.003 | 10.5 | 0.002 | 0.9 | 0.3 |
Mass of secondary ramets | 4.2 | 0.04 | 23.8 | 0.001 | 26.8 | <0.001 | <0.1 | 0.9 | <0.1 | >0.9 | <0.1 | 0.9 | 2.6 | 0.11 |
Mass per secondary ramet | 10.1 | 0.002 | 34.9 | <0.001 | 49.9 | <0.001 | 0.7 | 0.4 | 0.7 | 0.4 | 0.6 | 0.4 | 1.8 | 0.2 |
No. of secondary ramets | 4.5 | 0.04 | 7.8 | 0.006 | 16.4 | <0.001 | 1.7 | 0.2 | 1.0 | 0.3 | 0.8 | 0.4 | <0.1 | >0.9 |
Mass of tertiary ramets | 9.0 | 0.003 | 29.6 | <0.001 | 22.8 | <0.001 | <0.1 | 0.8 | 0.3 | 0.6 | 2.9 | 0.09 | 12.9 | 0.001 |
Mass per tertiary ramet | 15.9 | <0.001 | 58.7 | <0.001 | 65.5 | <0.001 | 0.5 | 0.5 | 1.0 | 0.3 | 0.4 | 0.5 | 9.5 | 0.003 |
No. of tertiary ramets | 2.3 | 0.14 | 10.5 | 0.002 | 19.9 | <0.001 | 0.3 | 0.6 | 0.3 | 0.6 | 1.2 | 0.3 | <0.1 | 0.9 |
Variable . | Fragmentation in first iteration (F1) . | Fragmentation in second iteration (F2) . | Fragmentation in third iteration (F3) . | F1 × F2 . | F1 × F3 . | F2 × F3 . | F1 × F2 × F3 . | |||||||
---|---|---|---|---|---|---|---|---|---|---|---|---|---|---|
F | P | F | P | F | P | F | P | F | P | F | P | F | P | |
High nutrient | ||||||||||||||
Total mass | 7.9 | 0.006 | 2.7 | 0.10 | 3.1 | 0.08 | <0.1 | 0.8 | 4.0 | 0.05 | 7.0 | 0.01 | 2.2 | 0.14 |
Mass per primary ramet | 22.9 | <0.001 | 20.9 | <0.001 | 42.1 | <0.001 | 0.5 | 0.5 | 0.7 | 0.4 | 1.6 | 0.2 | 4.9 | 0.03 |
Mass of secondary ramets | 1.0 | 0.3 | 12.7 | 0.001 | 25.9 | <0.001 | 0.9 | 0.3 | <0.1 | >0.9 | 0.4 | 0.5 | 0.2 | 0.7 |
Mass per secondary ramet | 5.5 | 0.02 | 28.0 | <0.001 | 43.8 | <0.001 | 0.3 | 0.6 | <0.1 | >0.9 | <0.1 | 0.9 | 0.4 | 0.5 |
No. of secondary ramets | 7.1 | 0.009 | 18.5 | <0.001 | 20.7 | <0.001 | 0.1 | 0.8 | <0.1 | >0.9 | 0.3 | 0.6 | 2.6 | 0.11 |
Mass of tertiary ramets | 2.7 | 0.10 | 23.6 | <0.001 | 41.2 | <0.001 | <0.1 | >0.9 | <0.1 | 0.8 | 0.3 | 0.6 | 0.1 | 0.8 |
Mass per tertiary ramet | 8.1 | 0.005 | 36.8 | <0.001 | 70.4 | <0.001 | 0.4 | 0.6 | 0.3 | 0.6 | <0.1 | >0.9 | 0.8 | 0.4 |
No. of tertiary ramets | 5.6 | 0.02 | 12.2 | 0.001 | 26.8 | <0.001 | 0.7 | 0.4 | 1.0 | 0.3 | 0.2 | 0.6 | 1.0 | 3.0 |
Low nutrients | ||||||||||||||
Total mass | 3.6 | 0.06 | 0.5 | 0.5 | 4.9 | 0.03 | 0.9 | 0.3 | 8.0 | 0.006 | 26.1 | <0.001 | 1.8 | 0.18 |
Mass per primary ramet | 17.5 | <0.001 | 28.5 | <0.001 | 48.2 | <0.001 | 0.3 | 0.6 | 9.4 | 0.003 | 10.5 | 0.002 | 0.9 | 0.3 |
Mass of secondary ramets | 4.2 | 0.04 | 23.8 | 0.001 | 26.8 | <0.001 | <0.1 | 0.9 | <0.1 | >0.9 | <0.1 | 0.9 | 2.6 | 0.11 |
Mass per secondary ramet | 10.1 | 0.002 | 34.9 | <0.001 | 49.9 | <0.001 | 0.7 | 0.4 | 0.7 | 0.4 | 0.6 | 0.4 | 1.8 | 0.2 |
No. of secondary ramets | 4.5 | 0.04 | 7.8 | 0.006 | 16.4 | <0.001 | 1.7 | 0.2 | 1.0 | 0.3 | 0.8 | 0.4 | <0.1 | >0.9 |
Mass of tertiary ramets | 9.0 | 0.003 | 29.6 | <0.001 | 22.8 | <0.001 | <0.1 | 0.8 | 0.3 | 0.6 | 2.9 | 0.09 | 12.9 | 0.001 |
Mass per tertiary ramet | 15.9 | <0.001 | 58.7 | <0.001 | 65.5 | <0.001 | 0.5 | 0.5 | 1.0 | 0.3 | 0.4 | 0.5 | 9.5 | 0.003 |
No. of tertiary ramets | 2.3 | 0.14 | 10.5 | 0.002 | 19.9 | <0.001 | 0.3 | 0.6 | 0.3 | 0.6 | 1.2 | 0.3 | <0.1 | 0.9 |
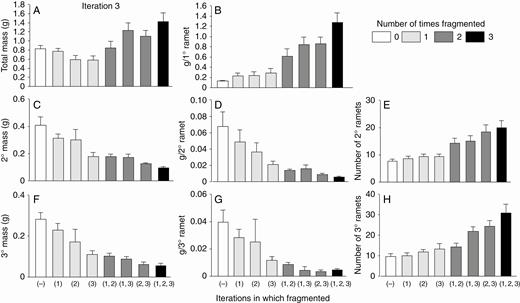
High-nutrient run: effects of the third iteration of fragmentation treatments on final total dry mass (mean + s.e.) of clonal fragments and mass, mass per ramet, and number of ramets in the parental (primary) ramet (1°), its offspring (secondary ramets, 2°) and their offspring (tertiary ramets, 3°). There was only one parental ramet, and thus mass per primary ramet equals mass of the primary ramets. The parental ramet was a secondary ramet in the second iteration.
Unlike the first and second iterations with high nutrients, the third iteration with high nutrients showed clear, positive effects of fragmentation on total mass of all ramets combined (Fig. 6A; Table 2). These effects partly resembled those seen in the second iteration with low nutrients in that total mass tended to increase with multiple fragmentation treatments due to compounding positive effects of fragmentation treatments on the primary ramet. However, unlike in the second iteration with low nutrients, a single round of fragmentation during the run with high nutrients did not increase total mass of all ramets combined.
With low nutrients (Fig. 7; Table 2), effects of the third iteration of fragmentation were mostly similar to effects with high nutrients. One difference was that final total mass of all ramets combined was about twice as great in plants fragmented in all three iterations as in plants fragmented in zero, one or two iterations (Fig. 7A). As in other cases, the positive effect of fragmentation on total mass was due to a positive effect on the primary ramet (Fig. 7B).
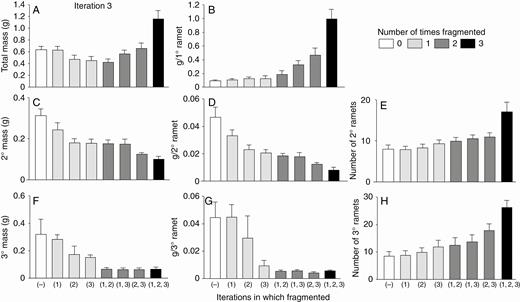
Low-nutrient run: effects of the third iteration of fragmentation treatments on final total dry mass (mean + s.e.) of clonal fragments and mass, mass per ramet, and number of ramets in the parental (primary) ramet (1°), its offspring (secondary ramets, 2°) and their offspring (tertiary ramets, 3°). There was only one parental ramet, so mass per primary ramet equals mass of the primary ramet. The parental ramet was a secondary ramet in the second iteration.
DISCUSSION
The results answered each of the three main questions positively. First, some effects of previous fragmentation persisted after fragmentation had ceased. With high nutrients, fragmentation in the first iteration decreased the individual and combined mass of new ramets produced in the second iterations. This was true even when plants were not fragmented in the second iteration. The simplest potential explanation would be that the starting, parental ramets in the second iteration had relatively low initial mass if they were from plants that had been fragmented and thus produced a relatively low mass of offspring. Persistence of effects of previous nutrient availability and herbivory between vegetative generations has similarly been attributed to provisioning of clonal offspring (Dong et al., 2019). However, the starting ramets in the second iteration themselves accumulated as much mass and produced as many offspring by the end of the iteration as did starting ramets from plants that had not previously been fragmented. This suggests that the persistence of effects of previous fragmentation was not simply due to lower overall growth but rather to persistent effects on allocation between parent and offspring. This alternative explanation is further supported by persistence of the same effects of first-iteration integration in the third iteration, even in plants not fragmented in either the second or the third iteration.
Previous work on clonal plants has identified other types of persistent effects of previous treatment of ramets that cannot be attributed to effects on provisioning. Gruntman and Novoplansky (2004) found that the tendency of ramets of Buchloe dactyloides to grow less root mass in the presence of a connected ramet of the same clone than in the presence of a connected ramet of a different clone partly persisted for at least 1 week after disconnection and then disappeared within 9 weeks. González et al. (2016) showed that certain drought regimes affected the response of detached vegetative offspring of Trifolium repens to subsequent drought regimes; the treatment with a demethylating agent largely eliminated these transgenerational effects, suggesting that they were mediated by epigenetic effects on DNA methylation. Epigenetic mechanisms are known to be an important source of transgenerational plasticity via sexual reproduction by seeds and may also underlie transgenerational effects via vegetative reproduction (Latzel and Klimešová, 2010; Herman and Sultan, 2011; Ezard et al., 2014; Verhoeven and Preite, 2014; Douhovnikoff and Dodd, 2015).
Second, all the main effects of fragmentation compounded when fragmentation was repeated in successive iterations. Fragmentation in each iteration increased mass of the parental ramet, decreased combined and individual mass of the offspring ramets, and increased the number of offspring ramets. At the end of the final, third iteration at high nutrients, each of these effects was generally greater in plants subjected to a greater number of fragmentation treatments, though more recent fragmentation tended to have a stronger effect. Dong et al. (2019) likewise found that effects of high nutrients compounded across two vegetative generations in Alternanthera philoxeroides, with greater effect of present than of previous nutrient level. Transmission of environmental effects across multiple generations via seeds has been documented (Galloway, 2005; Riis et al., 2010; Groot et al., 2016), but no previous study appears to have tested for either persistence or compounding of environmental effects across more than two vegetative generations.
The effects of fragmentation within iterations largely resemble those seen in comparable studies. For example, other studies on aquatic, clonal plants have reported that fragmentation decreased mass per offspring and increased number of offspring (Dong et al., 2012; Wang et al., 2014, 2016; Han et al., 2018) and increased parental mass (Wang et al., 2014, 2016), sometimes with a negative effect on combined mass of offspring (Wang et al., 2016) and sometimes with no effect (Dong et al., 2012; Han et al., 2018). This is consistent with net resource transfer from parent to connected offspring even in homogeneous conditions (Dong et al., 2015), and with signalling for apical dominance between connected ramets.
Third, persistence and compounding of effects of fragmentation were greater when resource levels were lower. Persistence of environmental effects across sexual generations in plants can be greater in more favourable environments (Galloway and Etterson, 2007; Fenesi et al., 2014; Dechaine et al., 2015) or in less favourable ones (Herman et al., 2012). Dong et al. (2019) showed greater compounding of effects of nutrient treatments at higher nutrients but attributed this to provisioning. More work is needed to tell if transgenerational effects of fragmentation in floating, clonal plants are generally more important in habitats with lower nutrient availability. It may be that effects via provisioning and effects via epigenetic changes will show different patterns.
We caution that, because it was not logically feasible to test effects of fragmentation at the two nutrient levels simultaneously, effects of nutrient level in this study might be confounded with differences in conditions, such as day length between the times of the test of each level. However, we are reasonably confident that the key effects of nutrient levels that we observed would have been similar if levels had been tested at the same time. The tests overlapped in time for one-third of their duration, and the results are readily interpretable as effects of nutrient levels.
Because only the fates of first-generation offspring were followed, it is not possible to completely assess how fragmentation affected total clonal performance across generations in P. stratiotes. It is still notable that fragmentation had no effect on combined final dry mass of ramets within the first iteration but had increasingly positive effects on total mass after the second and third iterations. This suggests that the absence of short-term effects of fragmentation on clonal performance does not mean that fragmentation will have no effect on clonal performance in the long term. The few previous experiments on fragmentation of aquatic, clonal plants that have measured short-term effects on clonal performance have yielded contrasting results. For example, fragmentation increased total mass in Ipomoea aquatica (Lin et al., 2012) and decreased it in A. philoxeroides, though only when grown with other species (Zhou et al., 2017a).
Persistent, compounding effects of fragmentation in floating, clonal plants could have important effects on the spread and density of these species, which include some of the most abundant and invasive wetland plants. Results here suggest that frequent disturbance and fragmentation can have increasingly positive effects on vegetative reproduction, which are likely to promote spread. Disturbance and nutrient levels can interact to produce different long-term effects on growth in mass, such that frequent disturbance at low but not high nutrient levels may increase total mass of clones after multiple vegetative generations. Prescriptions for management of invasive clonal species may thus be different in habitats with different disturbance and nutrient levels. More generally, results indicate that integration via resource transport and signalling between connected ramets, a key physiological property of clonal plants, underlies their capacity for invasiveness and abundance.
ACKNOWLEDGEMENTS
F.H.Y. and D.L.D. conceived the idea for the experiment; M.O.A. performed the experiment and collected the data; M.O.A., F.H.Y. and P.A. performed data analyses; M.O.A. drafted the manuscript with input from F.H.Y.; and P.A. revised the manuscript with input from F.H.Y and M.O.A.
FUNDING
This study was supported by the National Natural Science Foundation of China (Grant No. 31870610, 31800341), the Ten Thousand Talent Program of Zhejiang Province (Grant No. 2018R52016) and the Joint Fund of Zhejiang Provincial Natural Science Foundation (Grant No. LTZ20C030001).