-
PDF
- Split View
-
Views
-
Cite
Cite
Ryan J Daniels, Steven D Johnson, Craig I Peter, Flower orientation in Gloriosa superba (Colchicaceae) promotes cross-pollination via butterfly wings, Annals of Botany, Volume 125, Issue 7, 4 June 2020, Pages 1137–1149, https://doi.org/10.1093/aob/mcaa048
- Share Icon Share
Abstract
Complex modifications of angiosperm flowers often function for precise pollen placement on pollinators and to promote cross-pollination. We explore the functional significance of the unusually elaborate morphology of Gloriosa superba flowers, which are divided into one hermaphrodite meranthium and five male meranthia (functional pollination units of a single flower).
We used controlled pollination experiments, floral measurements, pollen load analyses and visitor observations in four populations of G. superba in South Africa to determine the breeding system, mechanism of pollination and role of flower in the promotion of cross-pollination.
We established that G. superba is self-compatible, but reliant on pollinators for seed production. Butterflies, in particular the pierid Eronia cleodora, were the primary pollinators (>90 % of visitors). Butterflies brush against the anthers and stigma during nectar feeding and pollen is carried on their ventral wing surfaces. Butterfly scales were positively correlated with the number of pollen grains on stigmas. We demonstrate that the styles were orientated towards clearings in the vegetation and we confirm that the highest proportion of initial visits was to hermaphrodite meranthia pointing towards clearings.
The flower morphology of G. superba results in effective pollen transfer on the wings of butterfly visitors. The style-bearing hermaphrodite meranthium of the flowers orientates towards open spaces in the vegetation, thus increasing the probability that butterflies land first on the hermaphrodite meranthium. This novel aspect of flower orientation is interpreted as a mechanism that promotes cross-pollination.
INTRODUCTION
The elaborate morphology of many angiosperm flowers is often a result of adaptations that ensure precise pollen placement on visitors during pollination (Harder and Johnson, 2009). Both floral morphology and the spatial arrangement of floral units, such as inflorescences, whole flowers and meranthia (components of a single flower that function as pollination units) can influence mating patterns (Barrett, 2003). Pollen transfer within flowers and among flowers on the same plant can have a deleterious effect on fitness due to pollen discounting (reduction in outcross siring opportunities) and ovule discounting (reduction in opportunities for cross-fertilized seed production) (Barrett, 2002). Both self-incompatible and self-compatible species can be detrimentally affected by self-pollination, but self-compatible species face additional risks of inbreeding depression following self-fertilization.
Plants have evolved numerous morphological mechanisms that reduce intra- and interfloral self-pollination (Bertin, 1993; Barrett, 2003; Routley et al., 2004). In approach herkogamy, for example, the stigma extends beyond the stamens, increasing the probability that approaching pollinators deposit pollen onto the stigma before collecting pollen (Brantjes and Bos, 1980; Barret, 2003). Protandry (a form of dichogamy in which the male phase follows the female phase) in racemose inflorescences functions to promote cross-pollination due to the acropetal behaviour of animals that land on lower female flowers and then move up the inflorescence, picking up pollen from younger male flowers (Harder et al., 2000; Routley et al., 2004, Jersakova and Johnson, 2007). Another key function of floral morphology is precise pollen placement on the pollinator’s body. Many species, orchids and milkweeds in particular, have evolved mechanisms for extraordinarily precise pollen placement (Peter and Johnson, 2006, 2013). Pollen depositions on the proboscis, head, thorax and abdomen of visitors are the most frequently observed systems (Ramírez, 1969; Dhileepan, 1992; Singaravelan and Marimuthu, 2004; Solomon and Aluri, 2011; Peter and Johnson, 2013, 2014; Tong and Huang, 2018). Pollen transfer via the wings of animals is a more unusual case that has gained some attention. Notable examples include the bat-pollinated Bauhinia pauletia (Fabaceae; Heithaus et al., 1974) and the carpenter bee-pollinated Mexacanthus mcvaughii (Acanthaceae; Holmqvist et al., 2005). Butterflies, particularly large butterflies, may be the best candidates for wing pollination as their wings flap relatively slowly and are corrugated with pronounced veins and scales, making them ideal for pollen placement (Greenewalt, 1960; Tercel et al., 2018). In general, large-winged insects can produce more force per beat, resulting in lower wing-beat frequencies (Tercel et al., 2018). Indeed, wing pollination appears to be reported most commonly in butterfly-pollinated species. For example, wing pollination has been described in Caesalpinia pulcherrima (Fabaceae; Cruden and Hermann-Parker, 1979), Clivia miniata (Amaryllidaceae; Kiepiel and Johnson, 2014), Rhododendron calendulaceum (Ericaceae; Epps et al., 2015) and Lilium martagon (Liliaceae; Corbera et al., 2018).
Because flowers have evolved reward and architectural traits to exploit the behaviour of pollinators, pollinators can often be predicted based on suites of floral traits or ‘pollination syndromes’ (Faegri and Van der Pijl, 1979; Willmer, 2011). Such butterfly-pollination syndrome (psychophilous) traits can be identified in many plants with wing pollination systems. Psychophilous traits are variable but the traditional description includes red, pink and mauve colours and flat-topped inflorescences or wide, flat petal margins to act as a relatively large landing platform (Faegri and Van der Pijl, 1979; Corbet, 2000; Willmer, 2011). Nectar is the sole reward for butterfly-pollinated flowers and is often hidden at the base of narrow, long tubes or spurs (Corbet, 2000). Flowers frequently have nectar guides and nectar has low volume and low solute concentration (Faegri and Van der Pijl, 1979, Willmer, 2011).
The genus Gloriosa (Colchicaceae) represents some of the most extraordinary examples of floral modification in the angiosperms. Lidstrom (1926), nearly a century ago, suggested that the complex flowers of Gloriosa superba may represent adaptations for outcrossing and wing pollination. The flowers are reflexed at the tip of the pedicel to create a nodding flower with a downward-pointing ovary and hypogynous stamens radiating perpendicularly to the axis of the ovary (Fig. 1A). The flower consists of six zygomorphic meranthia, one of which is hermaphroditic while the rest are staminate. The sexual states of the meranthia arise because the style bends sharply to one side so that the tri-lobed stigma is positioned between two of the six anthers.
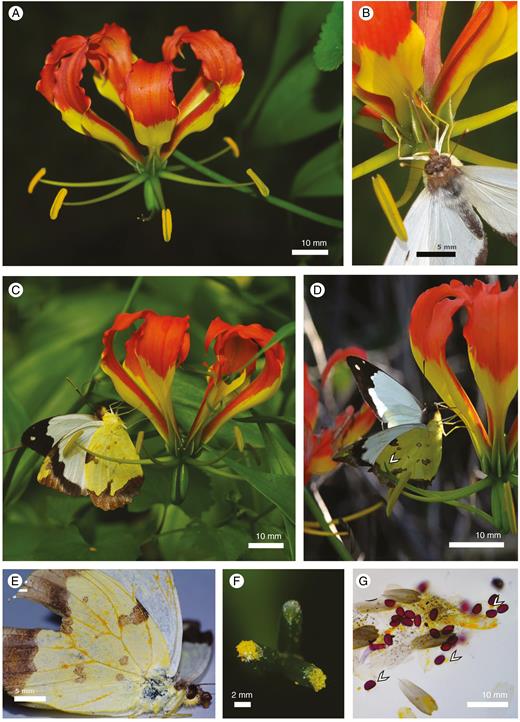
(A) Gloriosa superba flowers consist of five male meranthia and a single hermaphrodite meranthium. (B) The likely primary pollinator of G. superba in southern Africa is the long-tongued butterfly Eronia cleodora (Pieridae), here positioned between stamens while probing the pouch nectary. (C) Anthers deposit pollen on the underside of both hind- and fore-wings of the butterflies. (D) Contact between the undersides of the wings and the three stigma tips (arrow). (E) Butterflies accumulate large loads of pollen on their wings, particularly along the veins. (F) Gloriosa superba stigma from panel (A) with abundant pollen and white Eronia cleodora wing scales deposited on two of the three stigmatic lobes. (G) Squash of a pollinated stigma showing the presence of pollen and wing scales as well as the abundant pollenkitt of each pollen grain (arrows).
Hingston (1930), working in India, was the first to document G. superba pollen being deposited on the wings of papilionid butterflies. More recently, Martins (2015) demonstrated the same for G. baudii (reported as G. minor) which is visited by pierid butterflies in Kenya. Le Roux and Robbertse (1997) observed multiple visitors to flowers of G. superba, including the swallowtail butterfly Papilio demodocus.
Detailed studies of the putative mechanism of transfer of Gloriosa pollen on the wings of butterflies and an adaptive explanation for the unusual floral morphology have, however, been lacking.
Based on previous reports of visitors to Gloriosa flowers and our preliminary field observations, we hypothesized that G. superba is pollinated by butterflies and that pollen is transferred on their wings. The style of G. superba often appears orientated towards open spaces in the vegetation. We hypothesized that this is a developmental adaptation that promotes cross-pollination by increasing the probability that butterflies will settle first on a hermaphrodite meranthium. To address these hypotheses, we posed the following questions. (1) Does G. superba depend on pollinator visits for seed production? (2) Is pollen carried on the wings of butterflies? (3) Is deposition of pollen on stigmas associated with deposition of butterfly wing scales? (4) Are floral advertising and reward traits consistent with the general syndrome of butterfly-adapted flowers? (5) Many flower visitors are likely to approach from open spaces, e.g. paths, and thus we ask whether flowers orientate with their styles facing open spaces in the vegetation? (6) Lastly, do butterflies initially settle preferentially on meranthia facing open spaces?
MATERIALS AND METHODS
Study species
Gloriosa superba is a cryptophyte with a perennial subterranean tuber and ephemeral, sparsely branched climbing stems which die back at the end of the growing season (Maroyi et al., 2013; Padmapriya et al., 2016). It occurs in tropical and subtropical South and South-East Asia and much of Africa from Ethiopia and Nigeria south to Port Alfred in the Eastern Cape province of South Africa (Maroyi et al., 2013; C. I. Peter, pers. obs.). The plants produce numerous flowers (average ≈6 per branch; Gupta and Raina, 2001) and cultivated plants may produce 7–58 flowers in a year (Chitra et al., 2010). Flowers are 5–12 cm in width and distinguished from those of the related species Gloriosa simplex by the highly crisped perianth margins (Maroyi, 2012a, b; Maroyi et al., 2013). The tepal colours change according to stage of anthesis, from light green (bud-opening) to crimson at the tip, yellow towards the mid-perianth and green at the base (stigma-receptive), and finally it turns entirely crimson (late post-pollination) (Gupta and Raina, 2001). Gupta and Raina (2001) suggested that there was an acropetal-like sequential opening to prevent geitonogamy, but this is unclear given the scrambling nature of the growth form. Nectaries are tube-like pouches, aligned along the midline of the adaxial surface of each tepal. A slit in the nectary runs towards the tepal base, making the ‘tube’ incomplete. In the Eastern Cape of South Africa flowering occurs during the austral summer between December and April.
Fruit are loculicidal, oblong capsules and each of the three locules produces ~20 seeds (30–77 seeds per fruit) (Chitra et al., 2010; Padmapriya et al., 2016).
Study sites
Data were collected from four natural populations in the Eastern Cape, South Africa. Observations were made at Morgan Bay (28.35° E, 32.70° S) over 6 d from mid-February to late March 2011 and 20 and 21 February 2012, and Breezy Point (28.35° E, 32.70° S) for 2 h on 16 February 2011, with a total of >100 plants observed. A further ~20 plants were observed at Dwesa Nature Reserve (28.83° E, 32.29° S) over 2 d on 19 and 20 February 2011. We also made a number of observations of ~50 plants that have naturalized in a botanical garden at Rhodes University, Grahamstown. Floral colour measurements were taken in the naturalized Rhodes University population (February 2011 and 2020) and a wild population at Kayser Beach (27.61° E, 33.20° S; 26 February). These sampling dates fall within the peak flowering period (Maroyi et al., 2013). While our sampling would not account for visitor turnover throughout the entire flowering period (e.g. Valverde et al., 2016), we aimed to identify the most important guild of visitors rather than specific species.
Controlled pollination experiments
To test whether G. superba plants are self-compatible as well as reliant on pollinators for seed set, flowers were identified in the Morgan Bay population and allocated to one of the following three treatments: bagged at the bud stage without further manipulation to test for autonomous seed production (n = 22); bagged and emasculated in the late bud stage before the anthers dehisced to test for agamospermy (n = 25); and left open and unmanipulated to test natural levels of fecundity (n = 23). The treatment trio were on the same plant where possible and within 2 m of one another. We performed a further two treatments: bagged at the bud stage and either manually self-pollinated to test for self-compatibility (n = 4); or manually cross-pollinated as a positive control (n = 5). Mature fruits were harvested shortly before dehiscence and scored for the presence of fruit and to determine how many ovules developed as seeds.
We tested for differences in fruit set between treatment pairs using Fisher’s exact test for count data as implemented in the R (3.5.1) stats package (R Core Team, 2018). Firstly, we tested for a difference between the bagged unmanipulated flowers versus the unbagged (open-pollinated) flowers as an indication of fruit production in the absence of pollination. Secondly, we tested for a difference between the bagged unmanipulated flowers versus the bagged emasculated flowers as an indication of agamospermy.
Differences in the mean number of seeds per fruit between treatments were analysed using a generalized linear model implemented in R using the package MASS (Venables and Ripley, 2018). This incorporated a negative binomial error distribution and log link function. The estimates and standard errors for seed set values were obtained by back-transformation of log values, resulting in asymmetrical standard errors. No seeds developed in the bagged emasculated treatment and since non-Gaussian generalized linear models require variance in each treatment group we added a value of 1 seed to a single observation in this treatment group to enable the model to run. This makes the test slightly more conservative (Zuur et al., 2009). Marginal means were estimated using the emmeans package (Lenth et al., 2019) in R. A post hoc pairwise Dunn–Šidák test was used to identify significant differences between treatments.
Floral advertising and reward traits
To assess flower colour, spectral reflectance was measured from the orange distal and yellow proximal adaxial surfaces of one tepal from six flowers from the Rhodes University naturalized population and seven flowers from the Kayser Beach wild population. Measurements were made using the Ocean Optics USB 2000 spectrophotometer as described by Peter and Venter (2017).
We measured the nectar pouch length to compare with the mean proboscis length of pollinators. Measurements were made on 12 flowers each from a different plant from the Rhodes University naturalized population and nine flowers from the Kayser Beach wild population. Measurements were made, on one meranthium from each flower, from the base of the nectary to the distal opening of the nectar pouch (0.1 mm accuracy) using electronic callipers.
Scanning electron microscopy was used to examine tepal epidermal structures, which may serve as structural nectar guides. Three tepals from three different flowers of the Rhodes University population were fixed in 3 % glutaraldehyde in 0.1 m phosphate buffer at pH 7 at room temperature for 3 h following the protocol of Fischer et al. (2012). The tissue was then dehydrated in a graded ethanol series (50, 60, 70, 80, 95 and 100 % for 10 min each) followed by critical-point drying, mounting with adhesive tape on stubs and sputter-coating in gold. Prepared specimens were imaged with a Vega SE Detector scanning electron microscope (Tescan, Czech Republic) at 20.00 kV.
We investigated the volume (standing crop) and solute concentration of nectar in flowers of the Morgan Bay population (n = 37). Nectar volume was measured using glass micropipettes and the solute concentration determined using a handheld refractometer (Atago N-50E, Japan). To determine whether there was nectar depletion through the day, we compared samples collected between 0700–0900 (n = 25 flowers) and 1300–1500 h (n = 12 flowers). We performed type III ANOVAs to test whether meranthium position and time of day, as fixed effects, influenced nectar concentration and volumes, as the response variables. The nectar volumes were transformed by taking the square root.
Pollen and pollenkitt
To explore possible mechanisms for pollen adhesion to the pollinators and to determine whether G. superba produces viscin threads as documented in other species depositing pollen on butterfly wings (Cruden and Hermann-Parker, 1979), we examined pollen exine structure using scanning electron microscopy. Pollen grains from freshly dehisced anthers (Rhodes University population, n = 3 flowers) were air-dried for 24 h before being mounted on adhesive tape. For comparison, a second sample of pollen grains were washed with carbon disulphide to remove the pollenkitt (Pacini and Hesse, 2005). Pollen grains were collected on glass coverslips and excess solvent was evaporated. The samples were then imaged as described above.
Pollinator observations and behaviour
We recorded the visitors to G. superba flowers (n = 83), measured the distance moved between the first and second flowers visited (n = 22) and determined the number of meranthia visited per flower (n = 63). The time spent per meranthium was estimated from voice recordings (n = 51).
We supplemented direct observations with reports from online citizen science platforms, including social media. We searched platforms LepiMAP (FitzPatrick Institute of African Ornithology, 2019) and iNaturalist using the keywords ‘Pieridae’ and ‘Papilionidae’ and, in the case of iNaturalist, geographically limited to ‘Africa’. Records (Pieridae, n = 22 642 and n = 3188; Papilionidae, n = 4813 and n = 1274 for LepiMAP and iNaturalist, respectively) were manually screened for images showing butterflies visiting flowers of Gloriosa species across Africa. Additional observations from the ‘Flora of Southern Africa’ Facebook group (https://www.facebook.com/groups/Floraofsouthernafrica/) were converted to entries on LepiMAP for this purpose (observations 2, 4 and 5 in Supplementary Data Fig. S1).
Pollen grains and wing scales were counted on stigmas to assess the association of pollen deposition with butterfly wing contact (as in Cruden et al., 1976; Kiepiel and Johnson, 2014). A greater number of scales deposited can be interpreted as a greater number of butterfly visits or as a more vigorous interaction of the butterfly wings with the stigma.
The stigmas of 27 flowers were removed and placed onto a 1-mm3 block of fuchsin gel (Beattie, 1971), which was then melted beneath a coverslip. Pollen and wing scales were counted along two orthogonal transects centred on the slide. The sum of the two transects is reported. The relation between scale and pollen numbers on stigmas was assessed using simple linear regression.
To confirm the presence of pollen grains on the visitors, butterfly visitors were photographed in the field at a resolution high enough to identify deposits of pollen (n = 9; e.g. Fig. 1) and a subset were collected at Morgan Bay and examined under a microscope (n = 5) to compare pollen on the wings with pollen from the anthers.
Flower orientation
Style orientation was hypothesized to promote outcrossing if stigmas were positioned such that they were most likely to be visited first by arriving pollinators bearing cross-pollen. We recorded style and pedicel orientation for flowers (Morgan Bay, n = 47). The angle of the style and pedicel in the horizontal was estimated relative to the nearest vegetation clearings. The shortest line to the focal flower from the vegetation on which the plants were climbing was considered 0° (Fig. 2). Angles were then determined anti-clockwise from this datum (line perpendicular to the face of the vegetation) when viewed from above (centred on the ovary). Concurrently, the compass orientation of the stigma was measured to test for a possible orientation to broader geographical phenomena, e.g. the ecliptic of the sun, rather than the local environment. We tested for directionality in the data with a Rayleigh test (Pewsey et al., 2013). The Rayleigh r value is the concentration of data in a direction and varies from 0 (randomly distributed) to 1 (strongly directional) (Zar, 1999; Pewsey et al., 2013).
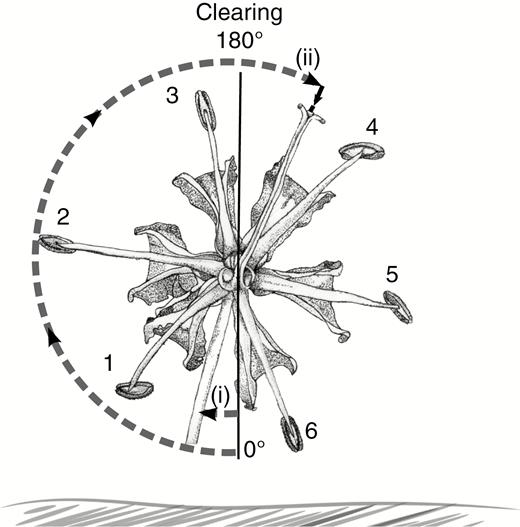
Gloriosa superba meranthia were identified clockwise from below. A perpendicular line was extended from the face of the vegetation out of which the flower was emerging to intersect the centre of the ovary, and this datum line was the line from which angles were measured. Stamens were numbered. We measured the angles from the datum to pedicle (i) and style (ii).
We tested the distribution of the data relative to the predicted direction of 180° for the style (towards the clearing) and 0° for the pedicel (outwards from the vegetation) using Watson’s two-sample test for homogeneity (Pewsey et al., 2013) and simulating 50 angles with a mean of 180 and 0°, respectively. The simulated variance matched the collected data. Analyses were done using the R package CircStats (Lund et al., 2017).
Visit order and stigma contact
To test whether butterflies disproportionately visit meranthia directed towards clearings when first approaching a flower, we observed the order of meranthia visited during each forage (n = 22). We considered flowers to be symmetrical on the axis of the pedicel, which was shown to be strongly directed towards the clearing (see the Results section) and pooled meranthia into three groups: outer meranthia, opposite the pedicel (numbered 3 and 4 and orientated towards the vegetation clearings, numbering as per Fig. 2); middle meranthia (2 and 5); and inner meranthia (1 and 6) orientated towards the vegetation and adjacent to the pedicel.
To test whether outer meranthia (3 and 4) are more likely to be visited on the initial alightment compared with the middle and inner meranthia (1, 2, 5 and 6), we used an intercept-only generalized estimating equation (Zeger and Liang, 1986). The response variable was coded as a binary for tepal position (outer = 1 and other = 0).
There is possible redundancy in visit order–tepal position combinations as there are two possible tepals for the outer position (3 and 4), and thus we may find that the initial two visits are to the outer tepals. To account for the redundancy, we used paired visit events in sequence (first and second tepal visited, second and third tepal visited). We could thus calculate the changes in mean probability of alighting on the outer tepal through the visit sequence. The generalized estimating equation allowed us to account for correlations between observations in each pair of visits from the same butterfly. Only the first flower visited for each butterfly was considered.
RESULTS
Controlled pollination experiments
The unbagged (open-pollinated) treatment produced significantly more fruit than the bagged but unmanipulated flowers (Fisher’s exact test, P < 0.001) (Table 1). The bagged and emasculated flowers produced no fruit while the bagged but unmanipulated flowers set some fruit (Fisher’s exact test, P < 0.001). Flowers that were self- or cross-pollinated all produced fruit, and produced similar numbers of seeds to those of open-pollinated flowers (Fig. 3) (Supplementary Data Table S1). Seed set following the bagged emasculated and bagged unmanipulated treatments was significantly lower than that arising from the pollinated or unbagged treatments (post hoc Dunn–Šidák correction test, P < 0.03).
Percentage of flowers that set fruit following various controlled pollination treatments on Gloriosa superba at Morgan Bay. See text for statistical analysis
Treatment . | Fruit set . |
---|---|
Unbagged | 100 % (n = 23) |
Bagged and emasculated | 0 % (n = 25) |
Bagged and not emasculated | 37 % (n = 22) |
Bagged and cross-pollinated | 100 % (n = 5) |
Bagged and self-pollinated | 100 % (n = 4) |
Treatment . | Fruit set . |
---|---|
Unbagged | 100 % (n = 23) |
Bagged and emasculated | 0 % (n = 25) |
Bagged and not emasculated | 37 % (n = 22) |
Bagged and cross-pollinated | 100 % (n = 5) |
Bagged and self-pollinated | 100 % (n = 4) |
Percentage of flowers that set fruit following various controlled pollination treatments on Gloriosa superba at Morgan Bay. See text for statistical analysis
Treatment . | Fruit set . |
---|---|
Unbagged | 100 % (n = 23) |
Bagged and emasculated | 0 % (n = 25) |
Bagged and not emasculated | 37 % (n = 22) |
Bagged and cross-pollinated | 100 % (n = 5) |
Bagged and self-pollinated | 100 % (n = 4) |
Treatment . | Fruit set . |
---|---|
Unbagged | 100 % (n = 23) |
Bagged and emasculated | 0 % (n = 25) |
Bagged and not emasculated | 37 % (n = 22) |
Bagged and cross-pollinated | 100 % (n = 5) |
Bagged and self-pollinated | 100 % (n = 4) |
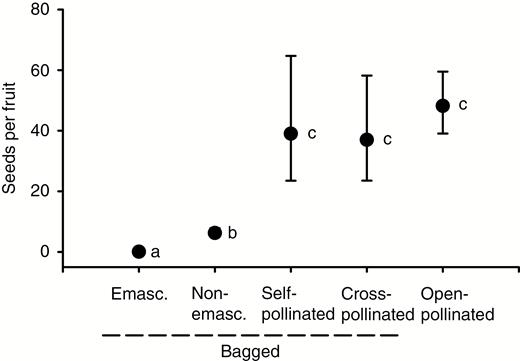
Effects of pollination treatment on seed production for Gloriosa superba from the Morgan Bay population. Values are mean (± standard error) number of seeds per fruit. Mean values that share a letter are not significantly different (post hoc Dunn–Šidák test). Sample sizes are provided in Table 1. Emasc. = emasculated.
Floral advertising and reward traits
Tepals from both the naturalized Rhodes University population and the Kayser Beach wild population were bicoloured with the distal halves visibly red and UV-reflecting, while the proximal tepal bases were yellow and UV-absorbing (Fig. 1 and Supplementary Data Fig. S2). The line marking the transition from red to yellow forms a ‘V’ with the apex pointing towards the nectary pouch (e.g. Fig. 1C). The tepal nectary pouches differed slightly but significantly between the Rhodes University and Kayser Beach populations (Welch two-sample t-test, t = 2.6, d.f. = 17.91, P = 0.02). Rhodes University pouch lengths were slightly longer (11.4 ± 2.1 mm, mean ± s.d.; n = 12) compared with the Kayser Beach flowers (9.6 ± 1.2 mm, mean ± s.d.; n = 9).
Paired longitudinal ridges run along the midlines of the tepals (Fig. 4D, E) and hairs on the fringe of the opening of the nectary slit (Fig. 4D) may function as mechanical guides.
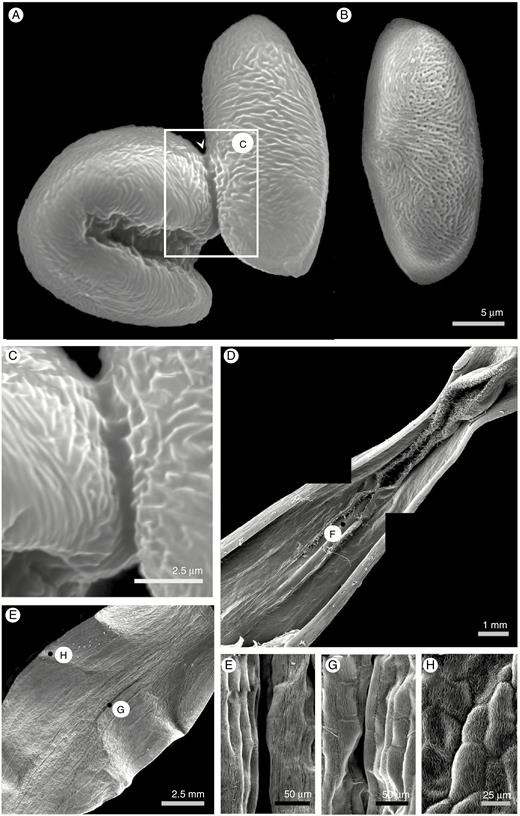
Scanning electron micrographs of pollen and tepals of Gloriosa superba. (A) Pollen grain. (B) Pollen grain treated with carbon disulphide to remove pollenkitt. (C) Interactions between pollenkitt lipids of two pollen grains in contact. (D, E) Composite low-power electron micrograph of the base (D) and middle third (E) of a single tepal showing the position of high-power views (F, G, H) demonstrating the surface texture of the tepal.
Nectar volumes were significantly different (P = 0.009; Table 2) between the morning (median = 1.75 μL, IQR = 0.77–2.82 μL, n = 25) and the afternoon (median = 0.94 μL, IQR = 0.40–1.36 μL, n = 12), but did not differ among meranthia for either morning or afternoon (P = 0.051) (Supplementary Data Fig. S3).
Influence of time of day and tepal position on volume and concentration of nectar (type III ANOVA). Indicated are the sum of squares (Sum sq.), d.f., F value and P value for the model with tepal position and time as main effects and interaction
Measure . | Predictor . | d.f. . | Sum sq. . | F value . | P value . |
---|---|---|---|---|---|
Volume | Time of day | 1 | 2.77 | 7.00 | 0.009 |
Tepal position | 2 | 2.40 | 3.03 | 0.051 | |
Interaction | 2 | 0.01 | 0.008 | 0.99 | |
Residuals | 117 | 46.29 | |||
Concentration | Time of day | 1 | 116 | 3.26 | 0.07 |
Tepal position | 2 | 92 | 1.31 | 0.27 | |
Interaction | 2 | 27 | 0.38 | 0.68 | |
Residuals | 95 | 3358 |
Measure . | Predictor . | d.f. . | Sum sq. . | F value . | P value . |
---|---|---|---|---|---|
Volume | Time of day | 1 | 2.77 | 7.00 | 0.009 |
Tepal position | 2 | 2.40 | 3.03 | 0.051 | |
Interaction | 2 | 0.01 | 0.008 | 0.99 | |
Residuals | 117 | 46.29 | |||
Concentration | Time of day | 1 | 116 | 3.26 | 0.07 |
Tepal position | 2 | 92 | 1.31 | 0.27 | |
Interaction | 2 | 27 | 0.38 | 0.68 | |
Residuals | 95 | 3358 |
Influence of time of day and tepal position on volume and concentration of nectar (type III ANOVA). Indicated are the sum of squares (Sum sq.), d.f., F value and P value for the model with tepal position and time as main effects and interaction
Measure . | Predictor . | d.f. . | Sum sq. . | F value . | P value . |
---|---|---|---|---|---|
Volume | Time of day | 1 | 2.77 | 7.00 | 0.009 |
Tepal position | 2 | 2.40 | 3.03 | 0.051 | |
Interaction | 2 | 0.01 | 0.008 | 0.99 | |
Residuals | 117 | 46.29 | |||
Concentration | Time of day | 1 | 116 | 3.26 | 0.07 |
Tepal position | 2 | 92 | 1.31 | 0.27 | |
Interaction | 2 | 27 | 0.38 | 0.68 | |
Residuals | 95 | 3358 |
Measure . | Predictor . | d.f. . | Sum sq. . | F value . | P value . |
---|---|---|---|---|---|
Volume | Time of day | 1 | 2.77 | 7.00 | 0.009 |
Tepal position | 2 | 2.40 | 3.03 | 0.051 | |
Interaction | 2 | 0.01 | 0.008 | 0.99 | |
Residuals | 117 | 46.29 | |||
Concentration | Time of day | 1 | 116 | 3.26 | 0.07 |
Tepal position | 2 | 92 | 1.31 | 0.27 | |
Interaction | 2 | 27 | 0.38 | 0.68 | |
Residuals | 95 | 3358 |
Nectar sugar concentrations (g/100 g) were 29.8 ± 5.9 (n = 25) and 32.0 ± 5.9 (n = 12) for morning and afternoon, respectively. Neither the time of day (P = 0.07) nor the tepal position (P = 0.27) were significant predictors of nectar concentration (Table 2, Supplementary Data Fig. S3).
Pollen and pollenkitt
Pollen grains of G. superba are an oblate monad of medium size (22 ± 2.8 μm in length, n = 26) with a sunken sulcus aperture when dry and a surface covered in apparently overlapping ridges, apparently reticulate or rugulate (Fig. 4A). No viscin threads were observed, but a well-developed pollenkitt was evident (Fig. 4A, B) forming a clear meniscus where adjacent pollen grains touch (Fig. 4C).
Pollinators and their behaviour
Butterflies were the most common visitors to G. superba; of these, Eronia cleodora subspecies cleodora (Pieridae) (Fig. 1C, D) made up 93.6 % of 79 butterfly observations, with only four visits by Papilio demodocus subspecies demodocus (Papilionidae) and one visit by Papilio dardanus subspecies cenea observed. Papilio nireus subspecies lyaeus (n = 10) were observed in the vicinity of G. superba, but none was seen to visit or inspect the flowers. Four southern double-collared sunbirds, Cinnyris chalybeus, were observed visiting flowers in the garden at Rhodes University (Supplementary Data Information S1). Citizen science records consistently show medium-sized butterfly species of the Pieridae visiting Gloriosa flowers throughout much of continental Africa (Supplementary Data Fig. S1). No visits by Papilionidae were recorded in the citizen science records.
As Eronia cleodora were the predominant visitors at our study sites, the following descriptions are based on their behaviour. Flying butterflies approached the flowers from the unvegetated ‘clearings’ and visited a meranthium. The tepal was grasped using their legs (Supplementary Data Video S1). The tepals are bounded on each side by stamens (or a stamen and the gynoecium for hermaphrodite meranthia) (Fig. 1A–D). Butterflies typically flutter their wings while probing for nectar, thus making repeated contact of the ventral surface of their wings with the anthers and hence picking up pollen loads (Supplementary Data Video S1). When visiting the hermaphrodite meranthium, butterflies bearing pollen on their wings may deposit pollen on the stigmas (Fig. 1A, B). Only in a few cases (6.3 %; 5 of 79) did the feeding behaviour at the flower differ (see Supplementary Data Information S1).
The time spent on a single meranthium was 4.4 ± 6.2 s (mean ± s.d.; IQR 1–3.5 s; median = 1s; n = 51). Sixty-six percent of butterflies were observed to only visit a single flower before moving out of sight (Fig. 5A). The median distance moved between the first and second flower was 100 cm (IQR 25–275 cm, n = 22). The median number of meranthia visited per flower per butterfly was 1 (IQR 1–2, n = 63), accounting for 58 % of the observations (Fig. 5B).
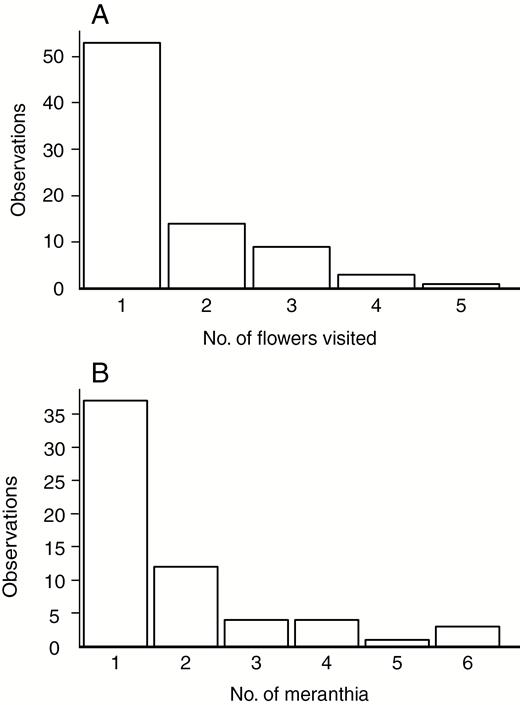
Frequency distribution of observations of the number of flowers visited per butterfly (n = 80) (A) and the number of meranthia visited per flower per butterfly (n = 63) (B) using only data from the first flower visited.
When feeding, butterflies extended their proboscides and repeatedly ‘stroked’ the tip of the bent proboscis along the tepal before inserting the tongue into the opening of the short nectary pouch (Supplementary Data Video S1). The tongue lengths of Eronia cleodora were 29.3 ± 1.2 mm (n = 5). Proboscides were significantly longer than the depth of the nectary pouches (mean pouch length: Kayser Beach, 9.5 mm; Rhodes University, 11.4 mm) (P < 0.001, Welch two-sample t-test; t = 30.6, d.f. = 8.5; and t = 22.7, d.f. = 13.3, respectively). There was thus a clear distance between the butterfly’s head and the nectary pouch as well as a sharp bend in the proboscis when feeding.
We found a significant positive correlation between the numbers of pollen grains and butterfly scales on the stigma tips (n = 27 flowers; Fig. 6).
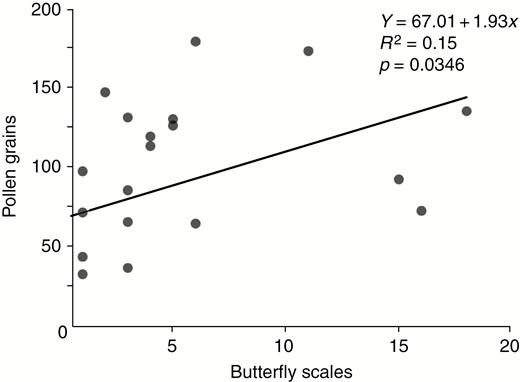
The number of pollen grains deposited on a stigma was positively correlated with the number of wing scales dislodged onto the stigma by the pollinator.
Flower orientation
The circular mean of the style orientation was 159° (n = 47, 25–75 % percentile 195°–135°) (Fig. 7A), i.e. away from vegetation (Rayleigh test statistic = 0.68, P < 0.0001). There was support for a significant difference compared with an outward-facing direction of 0° (Watson’s two-sample test statistic = 1.78, P < 0.001), but not from a direction of 180° (0.11, P = 0.1). The mean pedicel orientation was 0° (n = 47, 25–75 % percentile 20°–330°), i.e. projecting from the vegetation (Fig. 7B), which is significantly different from random distribution (Rayleigh test statistic = 0.701, P < 0.0001) and different from the inward-facing direction of 180° (Watson’s test statistic = 1.76, P < 0.001) but not an outward-facing direction of 0° (Watson’s test statistic = 0.01, P > 0.1).
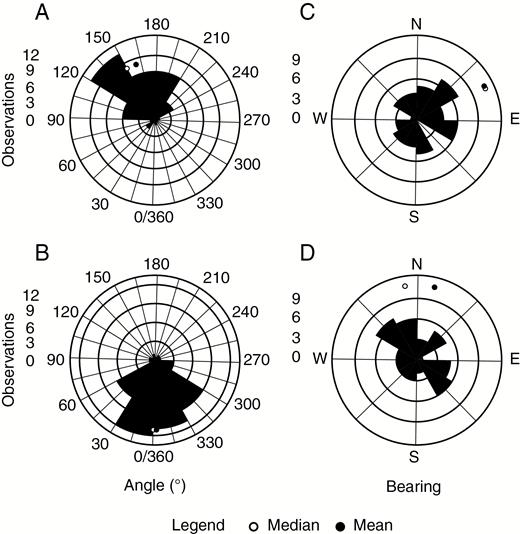
Observations of the orientation of styles (A, C) and pedicels (B, D) of Gloriosa superba. Presented are two sets of measurements: (A, B) angle relative to a line perpendicular to the face of the vegetation from which the flower is emerging (0°) with the clearing directly opposite (180°) and (C, D) relative to the geographical bearings.
Tests of the measurements relative to east (a measure of the sun’s path) indicated that neither the style nor the pedicel was different from a random distribution (Rayleigh test statistic <0.13, P = 0.4 and 0.08, P = 0.7, respectively) (Fig. 7C, D).
Visit order and stigma contact
The largest proportions of the first and second visits were to outer meranthia facing the clearing (meranthia 3 and 4) (Fig. 8) and the lowest proportions to inner and middle meranthia facing the supporting vegetation (meranthia 1, 2, 5 and 6). From the third visit event onwards middle and inner meranthia were more frequent.
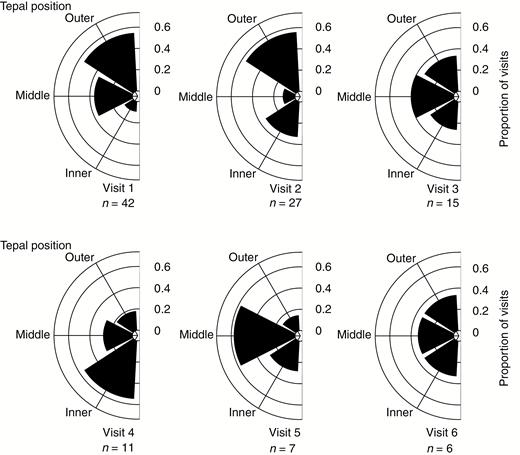
Proportional contribution of each tepal position (inner, middle and outer) to visits in sequence (first six visits) shown as a rose diagram. Data include only the first flower visited by each butterfly with tepals grouped as follows: outer (3 and 4); middle (2 and 5); and inner (1 and 6), as in Fig. 2. The sample size of the tepal-visit observations (n) for each visit event is indicated. Height of each bar indicates the proportion represented. Proportions marked by arches.
The probability of alighting on the outer tepals was 0.82 (95 % CI 0.7–0.9), which is significantly greater than the predicted 0.33 probability if meranthium choice was random (Fig. 9).
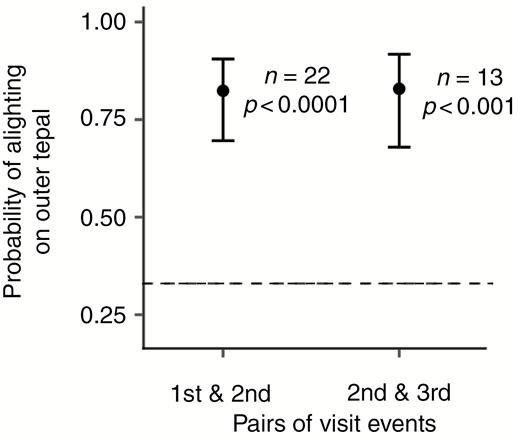
Change in probability of alighting at the outer meranthia between pairs of sequential visit events. The dashed line indicates the predicted probability if tepal choice is random. Shown are sample sizes (n) and P values.
DISCUSSION
This study shows that Gloriosa superba possesses exquisite floral adaptations for transfer of pollen on the wings of butterflies. From field observation and collected specimens we observed pollen on the underside of the wings of the visiting butterflies, particularly the pierid E. cleodora. We further documented clear evidence of regular contact of their wings with the stigma and anthers when feeding and we found a positive correlation of pollen grains and wing scales on stigmas (Fig. 6). This supports the likely role of butterflies in pollen transfer, as has been shown for other butterfly-pollinated species (Kiepiel and Johnson, 2014; Epp et al., 2015). Citizen science records (Supplementary Data Fig. S1) further indicated that pierid butterflies of similar sizes may be important throughout Africa as they have been frequently observed on flowers of G. superba. Pierids have similarly been reported as visitors to G. baudii in Kenya (Martins, 2015) and G. superba in India (Hingston, 1930). The present study thus confirms earlier suggestions that some Gloriosa species are specialized for wing pollination by butterflies (Lidstrom, 1926; Hingston, 1930; Mamatha et al., 1993; Martins, 2015).
Wings are necessarily dynamic structures and thus adaptations to enhance the attachment of pollen are essential for effective transfer. In Caesalpinia pulcherrima and the putatively butterfly-pollinated Delonix reginae, pollen is held together by viscin threads and deposited on the wings in clumps (Cruden and Hermann-Parker, 1979; Cruden and Jensen, 1979). An alternative to viscin threads, we propose here, is ‘stickiness’ provided by the pollenkitt, the outermost hydrophobic lipid layer of the pollen grain. Pollenkitt has numerous possible functions. In entomophilous species there is increased viscosity of the pollenkitt and an increased presence of pollenkitt on the exine, while in anemophilous species pollenkitt is often between columellae instead of the exine (Pacini and Hesse, 2005). This is likely related to its role in adhesion to the pollinator. The adhesive force between neighbouring pollen grains in G. superba, possibly facilitated by the exine microstructures observed, may cause clumping, which could collectively contribute to adhesion to the wings. Abundant pollenkitt is similarly proposed by Corbera et al. (2018) as a key trait of the wing-pollination by butterflies in Lilium martagon.
The flowers of G. superba consist of six segments, each allowing the visitor the opportunity to remove copious amounts of pollen (Hingston, 1930; Martins, 2015). Since the flower has a single stigma there is a great risk of selfing and sexual interference. The placement of the style is thus of great importance for fitness. We demonstrate that the hermaphrodite, style-bearing meranthium is orientated away from the surrounding vegetation upon which the scrambling growth form of G. superba is supported. Thus, the hermaphrodite meranthium is likely to receive the first visit by butterflies approaching from open spaces. We found that butterflies have a significantly greater than chance probability of their initial visit being to the ‘outward’-pointing meranthia. Furthermore, the styles were not directed based on the sun’s path (east–west) but instead were projected towards clearings, i.e. the likely direction of incoming visitors. Directional growth of the style in response to light, as has been demonstrated for Lilium martagon (Brantjes and Bos, 1980; and see Corbera et al., 2018), may be the mechanism by which this is achieved. We argue that this developmental trait may maximize cross-pollination as subsequently visited male meranthia can load pollen onto butterfly wings with reduced risk of causing sexual self-interference.
By presenting few open flowers with small amounts of nectar, G. superba may further discourage multiple foraging bouts on a single plant, thus lowering the risk of self-pollination. Insect foraging efficiency depends on handling time, time spent travelling between flowers and the cost of moving between sources on a single flower. Inter-flower travel costs are relatively low for massed flowers such as those in compact inflorescences, as these allow foragers to move between flowers by walking, rather than flying (Corbet, 2000). Gloriosa superba produces solitary flowers with a long pedicle and often only a few flowers per plant are produced, with sequential opening of flowers to prevent overlap in receptivity (Gupta and Raina, 2001). There are only six nectaries per flower and a flight is needed to move between adjacent meranthia.
In addition to the energetics of intrafloral movement, the likelihood of finding nectar has been shown to influence how far visitors travel between flower inspections (Kadmon and Shmida, 1992). We found evidence for nectar depletion between morning and midday and this may also discourage feeding from multiple tepals. In the early morning all tepals have sizeable volumes of nectar, such that a foraging butterfly may visit all six tepals. Later in the day, most tepals would have reduced nectar. This depletion would discourage further probing after the initial attempt, considering the cost of moving between tepals. From our data, the clear majority (>58 %) of butterflies only visited a single tepal and a single plant per patch. We argue that this is the outcome of the cost of foraging and the likelihood of finding depleted nectar. The high frequency of single visits also places emphasis on the importance of style orientation to intercept incoming visitors. Collectively these traits should minimize the possibility of geitonogamy.
Self-compatible plant species may experience a double cost of self-pollination: the waste of gametes and the associated lost reproductive opportunities; and the subsequent inbreeding depression in offspring (Barrett, 2002). The cost can explain the evolution of elaborate floral structures to reduce the likelihood of self-pollination and thus sexual self-interference (Barrett, 2002). Our controlled pollination experiments show that G. superba is self-compatible but that it requires pollen vectors for seed production (Fig. 3). The results of these breeding system experiments are similar to those previously published (Mamatha et al., 1993; Martins, 2015, but see Venudevan et al., 2011), and our results were suggestive of greater seed-set for cross-pollination, as has been confirmed for G. superba (Venudevan et al., 2011), G. rothschildiana (Krause, 1986) and G. baudii (Martins, 2015). There is thus a demonstrated need for floral visitors and a conceivable driver for the avoidance of selfing.
The overall colour signals of G. superba are similar to those of other butterfly-pollinated flowers (Cruden and Hermann-Parker, 1979; Kiepiel and Johnson, 2014; Martins, 2015) including the red-yellow morph of Lantana camara (Weiss, 1997), Caesalpinia pulcherrima (Cruden and Hermann-Parker, 1979), Clivia miniata (Kiepiel and Johnson, 2014) and Asclepias curassavica (Bierzychudek, 1981). We propose that the bi-colour abaxial surface may function as a visual guide for probing butterflies with the ‘V’ pattern point-down along the tepal midline to the nectary. Similar orange–yellow colour contrasts play a functional role in some colour morphs of L. camara/ L. camara/ (Weiss, 1997). The orange distal section of the tepal shows UV reflectance of ~40 % and several butterfly species, including Pieridae, are known to perceive light in the UV spectrum (Tovée, 1995). For example, light reflected off wings is used as sexual signals for mating (Obara and Hidaka, 1968). Further support for the importance of the colour in G. superba is given by the rapid post-pollination colour changes documented by Gupta and Raina (2001).
The nectar volumes were small (0.4–2.8 µL) and nectar concentrations (~30 %) intermediate, in agreement with many other butterfly-pollinated plants (Kingsolver and Daniel, 1979; Solomon and Aluri, 2011; Cahenzli and Erhardt, 2012; Martins, 2015). The solute concentrations are within the theoretical expectations for the broad optimum for energy transfer efficiency for most butterflies (15–30 %) (Kingsolver and Daniel, 1979).
The nectary pouch lengths we measured (~9–12 mm) should exclude the commonly reported short-tongued insect pollinators such as hymenopterans and dipterans in favour of visitors with longer tongues. Tongue length is correlated with body size in butterflies (Corbet, 2000), suggesting that medium to large butterflies are best adapted to accessing the nectar of G. superba flowers, as was confirmed by observations and citizen science records. The exclusion of the common short-tongued pollinators would reduce the rate of depletion and ensure a larger standing crop for larger pollinators, who are best suited morphologically to touch the anthers and stigma. The distance between anthers should make small insects ineffective at picking up pollen while collecting nectar. Since the two activities are strongly linked, it seems unlikely that visitors with wingspans smaller than 3–6 cm are effective pollinators (Lidstrom, 1926; Mamatha et al., 1993).
Feeding behaviour at the tepal involved butterflies stroking the tepal with their proboscides in search of the nectary (Supplementary Data Video S1). Longitudinal grooves on the tepal (described as protruding veins and midrib; Maroyi, 2012a) and the hair-like structures along the lip of the pouch slit (Fig. 4) likely function as tactile guides. Such guides have been demonstrated to be important in assisting pollinators in locating the reward and learning to handle new flowers (Goyret, 2006). These guides, in conjunction with visual guides, may be particularly important for long-tongued pollinators, which have to deftly manoeuvre the tip of their tongues into the narrow opening of the nectary.
Other members of the genus Gloriosa share many of the traits described here. The lengths of the filaments and style are usually long, but their orientation varies between species (Maroyi et al., 2013). Not all species have the reflexed tepals, a bent stigma, nectary hairs or the same colouration. There is also a possible case of a floral reversion between G. baudii and Gloriosa revoilii (formerly Littonia) (Vinnersten and Manning, 2007; Maroyi, 2012b). An investigation into the pollination strategies within the genus will provide important information for understanding evolutionary drivers for wing pollination.
Members of the Northern Hemisphere genus Lilium show some convergence with Gloriosa [e.g. Lilium martagon (Corbera et al., 2018) and, notably, Lilium humboldtii]. They also have a nodding flower, reflexed tepals and orange–red colour and produce copious amounts of pollen. Another species, Lilium philadelphicum, has structurally similar flowers, including the presence of a nectary slit along the tepal. We found online photographic records of Papilio species visiting these flowers with evidence of pollen borne on their wings. Comparative studies of floral morphology and pollination systems across such allied genera could shed further light on the evolutionary pathways for such traits.
Conclusions
The flowers of G. superba clearly represent a case of adaptation for pollination via the wings of butterflies. We have argued that elaborate floral architecture in G. superba manipulates the behaviour of visiting butterflies to reduce selfing and promote cross-pollination. Flowers project out of the surrounding vegetation and the stigma orientates towards clearings to intercept butterflies, resulting in a high proportion of initial visits to the outward-pointing meranthia, which is likely to lead to outcrossing.
SUPPLEMENTARY DATA
Supplementary data are available online at https://dbpia.nl.go.kr/aob and consist of the following. Figure S1: citizen science records of species of ‘white’ butterflies (Pieridae) visiting flowers of Gloriosa at various sites throughout sub-Saharan Africa.
Figure S2: reflectance spectra for tepals of Gloriosa superba. Figure S3: changes observed in nectar concentration and volume with time of day and tepal position. Table S1: post hoc pairwise Dunn–Šidák test for differences between breeding experiment treatments. Information S1 brief description of the behaviour of visitors to Gloriosa superba flowers not discussed in the main text. Video: demonstration of butterfly behaviour at Gloriosa superba flowers visitor behaviour.
FUNDING
This work was funded by Rhodes University and the South African National Research Foundation (NRF), and R.J.D. was supported by a Claude Leon Foundation Scholarship and a Rhodes University Council Loan.
ACKNOWLEDGEMENTS
We thank Gareth Coombs and Goetz Neef for assistance with fieldwork and Sean and Robyn Rohm at Yellowwood campsite for the camping facilities. The contribution by citizen scientists listed in Supplementary Data Fig. S1 is gratefully acknowledged. The authors declare no conflict of interests.