-
PDF
- Split View
-
Views
-
Cite
Cite
Aretuza Sousa, Julia Bechteler, Eva M Temsch, Susanne S Renner, Different from tracheophytes, liverworts commonly have mixed 35S and 5S arrays, Annals of Botany, Volume 125, Issue 7, 4 June 2020, Pages 1057–1064, https://doi.org/10.1093/aob/mcaa027
- Share Icon Share
Abstract
Unlike other nuclear genes in eukaryotes, rDNA genes (5S and 35S loci) are present in numerous copies per cell and, when stained, can therefore provide basic information about genome organization. In tracheophytes (vascular plants), they are usually located on separate chromosomes, the so-called S-type organization. An analysis of 1791 species of land plants suggested that S-type arrays might be ancestral in land plants, while linked (L-type) organization may be derived. However, no outgroup and only a handful of ferns and bryophytes were included.
We analysed genome sizes and the distribution of telomere, 5S and 35S rDNA FISH signals in up to 12 monoicous or dioicous species of liverworts from throughout a phylogeny that includes 287 of the 386 currently recognized genera. We also used the phylogeny to plot chromosome numbers and the occurrence of visibly distinct sex chromosomes.
Chromosome numbers are newly reported for the monoicous Lejeunea cavifolia and for females of the dioicous Scapania aequiloba. We detected sex-related differences in the number of rDNA signals in the dioicous Plagiochila asplenioides and Frullania dilatata. In the latter, the presence of two UU chromosomes in females and additional 5S-35S rDNA loci result in a haploid genome 0.2082 pg larger than the male genome; sex-specific genome differences in the other dioicous species were small. Four species have S-type rDNA, while five species have mixed L-S rDNA organization, and transitions may have occurred multiple times, as suggested by rDNA loci not being conserved among closely related species of Pellia. All species shared an Arabidopsis-like telomere motif, and its detection allowed verification of the chromosome number of Radula complanata and chromosome rearrangements in Aneura pinguis and P. asplenioides, the latter also showing sex-specific interstitial telomere repeats.
The S and L rDNA arrangements appear to have evolved repeatedly within liverworts, even in the same species. Evidence for differential accumulation of rDNA between the sexes so far is limited.
INTRODUCTION
Eukaryotic cells contain millions of ribosomes, and the DNA loci coding for ribosomal RNA (rRNA) are correspondingly abundant. They are organized in repeat units occurring on species-specific locations on the chromosomes. In plants, the most important are the 35S loci (encoding 18S, 5.8S and 26S rRNA genes) and the 5S loci (encoding 5S rRNA), which in vascular plants are usually physically separated from each other. This is called the separate or S-type arrangement (Garcia et al., 2017). Physical linking of 35S and 5S rDNA, the so-called linked or L-type arrangement, is considered rare. Thus, of 2949 karyotypes from 1791 species in 86 families of land plants, 87 % have the S-type organization, while 13 % have the L-type arrangement (Garcia et al., 2017). This species sampling, however, included only a handful of ferns and bryophytes, and no algae, the outgroup to land plants. An earlier study that had analysed ribosomal DNA sequences from at least two members of each of the major lineages of green and red algae, bryophytes and tracheophytes, by contrast had postulated that the S-type organization might be ancestral in land plants (Wicke et al., 2011). However, without FISH (fluorescence in situ hybridization) analyses the physical organization of ribosomal DNA cannot be seen. Thus, of ~7000 species of liverworts, just five have been studied in terms of organization of their 5S and 35S rDNA sites, namely the three dioicous species Marchantia polymorpha, Pellia endiviifolia and Pellia neesiana (male and female individuals) and the monoicous Pellia epiphylla and Pellia borealis (Sone et al., 1999; Nakayama et al., 2001; Fujisawa et al., 2003; Orzechowska et al., 2010, 2018).
Analysing the organization of rDNA loci in liverwort chromosomes is relevant not only to increasing our knowledge of land plant ribosomal DNA organization but also for understanding the genetic make-up of plant sex chromosomes. Sex chromosomes are pairs of homologous chromosomes that bear an organism’s main sex-determining locus and in which recombination is suppressed. Recombination suppression entails less efficient purifying selection on the non-recombining regions, which can lead to an accumulation of repetitive DNA, transposable elements and organellar DNA (reviewed in Bachtrog, 2008, 2013). Theory therefore predicts the accumulation of rDNA in sex chromosomes, but with important differences between haploid-dominant and diploid-dominant organisms. As in all land plants, bryophyte meiosis occurs in sporangia (in seed plants called pollen sacs and ovules), which form inside minute sporophytes that develop from fertilized egg cells. The diploid nuclei of bryophyte sporophytes therefore all contain one set of paternal and one of maternal chromosomes. Bryophyte sex chromosomes should thus both have the same level of recombination suppression, while in diploid-dominant organisms one of the two sex chromosomes will find itself in the company of a homologous sex chromosome with which it recombines at meiosis at least half the time. This led Bull (1978, 1983) to suggest that the sex chromosomes of bryophytes (now called U and V chromosomes; Bachtrog et al., 2011) should have identical evolutionary trajectories and that ‘in fact, it is merely convention to refer to the female sex chromosome as the X and the male sex chromosome as the Y, because there is no longer a heterogametic and homogametic sex’ (Bull, 1983, p. 262). Theory on sex chromosome evolution in haploid-dominant organisms, however, is little developed (Immler and Ottto, 2015), and even basic microscopic differences between male and female karyotypes, for example in repetitive DNA accumulation, are insufficiently known.
We here contribute data on rDNA accumulation and organization in monoicous and dioicous liverworts, using 5S, 35S and telomere FISH probes, combined with C-value measurements, to characterize the karyotypes of nine dioicous and three monoicous species placed in a large phylogeny (for a few species we lack either FISH or genome size data for both sexes). Questions we wanted to answer concerned (1) the occurrence of L-type or S-type rDNA organization in liverworts, (2) the extent to which male and female karyotypes differ in their ribosomal DNA organization, and (3) the possible occurrence of interstitial telomere repeats (ITRs) on sex chromosomes, because this might be a footprint of recent chromosome rearrangements (Sousa and Renner, 2015 and references therein).
MATERIALS AND METHODS
Plant material
Liverwort specimens were collected between April and November 2018 at three sites in Bavaria, Germany. Plants were identified under a stereomicroscope with the help of specialized literature (Paton, 1999; Frahm and Frey, 2004), followed by DNA sequencing and checking their phylogenetic placement in phylogenies based on standard DNA markers. Species of interest were cultivated under two conditions: either in an oven with a constant temperature of 15 °C and 8 h of light exposure (floodlight 6000–7000 K, cool colour) or in a cold room with natural light coming through windows and natural variation of temperature. All species, with their taxonomic authorities, herbarium vouchers and GenBank accession numbers, are listed in Supplementary Data Table S1.
DNA isolation and phylogenetic analyses
Gametophyte tissue was isolated from fresh material using the Macherey Nagel NucleoSpin Plant II kit. The PCR amplification protocols for the nuclear 26S gene, the plastid markers rbcL, rps4 and psbT and the mitochondrial marker nad1 followed Laenen et al. (2014). Sequencing primers were the same as used for the PCR. The software PhyDE version 0.9971 (Müller et al., 2010; http://www.phyde.de/) was used for generating consensus sequences and for manually aligning our new sequences into the data matrix of Laenen et al. (2014). The dataset includes at least one species from 287 of the 386 currently recognized liverwort genera (Söderström et al., 2016) and is based on the plastid loci atpB, psbA, psbT, rbcL and rps4, the nuclear locus 26S and the mitochondrial loci rps3 and nad1. The concatenated matrix comprised 317 species and 7617 characters after excluding sections of ambiguous alignment. The tree was rooted on mosses (Puttick et al., 2018; de Sousa et al., 2018).
Maximum likelihood (ML) analyses were conducted on the concatenated matrix using RAxML version 8.2.10 (Stamatakis, 2014) with the GTR + Γ model, with ten independent ML searches run in combination with multiparametric bootstrapping using the autoMRE function (Pattengale et al., 2010). The resulting tree was visualized in FigTree 1.4.3 (Rambaut, 2006–2009). Nodes with bootstrap values ≥95 % were regarded as well supported.
Chromosome preparation and FISH probes
Young thalli or apical meristems were collected in the morning, fixed in freshly prepared 3:1 (v/v) ethanol/glacial acetic acid at room temperature for 4 h, transferred to 70 % ethanol, kept at room temperature overnight, and afterwards stored at −20°C until use. For chromosome preparations, we used the dropping method described in Sousa et al. (2016), but reduced the enzymatic digestion period to 1 h 20 min. The quality of spreads was checked microscopically after counterstaining slides with DAPI (2 µg mL−1) and mounting in Vectashield (Vector). Slides with at least five metaphases were selected for FISH. Before FISH, DAPI was removed from the slides by first incubating them in freshly prepared 3:1 (v/v) ethanol/glacial acetic acid at room temperature for 30 min and then in 100 % ethanol for 2 h at room temperature or at 4 °C overnight.
Our FISH protocol was modified from Orzechowska et al. (2010), Sousa et al. (2013) and Aliyeva-Schnorr et al. (2015). As a pretreatment, the slides were placed in a Coplin jar containing 2 × SSC for 5 min, transferred to a Coplin jar containing 45 % acetic acid for 5 min and washed additionally for 10 min in 2 × SSC. Chromosomes were fixed in 4 % formaldehyde (in 2 × SSC) for 10 min, washed three times for 4 min in 2 × SSC, dehydrated in a series of 70, 90 and 100 % ethanol for 2 min each, and then dried at room temperature for at least 1 h before the hybridization step. The hybridization mix consisted of 50 % formamide (w/v), 2 × SSC, 10 % dextran sulphate (w/v) + 0.25 µg µL−1 salmon sperm DNA and 90–200 ng of labelled probe. The hybridization mix was denatured at 80 °C for 10 min and immediately cooled on ice for 10 min. We then added 10–15 µL of the mix to each slide, covered it with a glass coverslip and sealed it with a plastic glue, and denatured the DNA for 5 min at 80 °C. Hybridization was carried out in a humid chamber at 37 °C for at least 20 h. Without adding the salmon sperm DNA in the hybridization mix, chromosomes completely dissolved after performing a re-hybridization; with its addition, we could keep the quality of the slides for three rounds of hybridization.
After hybridization, the plastic glue surrounding the coverslips was removed and slides were placed in a Coplin jar containing 2 × SSC until the coverslips fell off. The slides were then washed once for 5 min in 2 × SSC at 42 °C, twice for 5 min in 10 % formamide in 0.1 °SSC at 42 °C, three times for 3 min in 2 × SSC at 42 °C, once for 5 min in 2 × SSC, and then in 4 × SSC/0.1 % (v/v) Tween 20 at room temperature. For digoxigenin and biotin detection, they were incubated in blocking buffer (5 % BSA in 2 × SSC) in a humid chamber for 30 min at 37 °C, followed by incubation with anti-digoxigenin-FITC conjugate (Roche) and streptavidin–Cy3 conjugate (Sigma) in 2 % BSA in 2 × SSC at 37 °C for 1 h. Excess antibody was removed by washing the slides three times for 5 min in 4 × SSC/0.1 % (v/v) Tween 20 at 42 °C. Slides were dehydrated in a series of 70, 90 and 100 % ethanol for 2 min each and left to dry for 5 min at room temperature, and chromosomes were then counterstained with DAPI (2 µg mL−1) and mounted in Vectashield (Vector). Images were captured with a Leica DMR microscope equipped with a KAPPA-CCD camera and the KAPPA software and optimized for best contrast and brightness using Adobe Photoshop CS3 version 10.0.
Slides first analysed with telomere and 5S rDNA probes were destained, and a second hybridization was performed with 35S rDNA to obtain a sequential staining with both markers in a single cell. The destaining protocol consisted of a short wash in 4 × SSC/0.1 % (v/v) Tween 20 to remove the coverslips, a wash of 1 h in the previous solution and a dehydration series of 70, 90 and 100 % ethanol for 2 min each, and finally slides were dried for at least 1 h before the hybridization step. All destaining washes were performed at room temperature.
We used standard telomere and 35S rDNA probes, except for a new 5S rDNA probe, which we generated because the standard probe from Beta vulgaris did not work in liverworts. To generate the new probe, we isolated DNA from Pellia endiviifolia and amplified its 5S rDNA using the primers 5′MPrrn5_for and 3′MPrrn5_rev (Sone et al., 1999). The 119-bp PCR product was confirmed to belong to the species after sequencing and was then cloned into TOPO®TA vector (Fisher Scientific, Schwerte, Germany). The resulting DNA fragment was PCR-labelled with DIG-11-dUTP or Biotin-16-dUTP.
Flow cytometry for C-value measurement
Fresh tissue samples of the species listed in Table 1 were wrapped in wet tissue and stored for up to 1 week at 4 °C. Measurements of C value followed the method of Temsch et al. (2010). Approximately 250 mg of gametophytic tissue was co-chopped in Otto’s buffer I (Otto et al., 1981) together with the internal standard Solanum pseudocapsicum (1C = 1.30 pg; Temsch et al., 2010) and subsequently filtered through a 30-µm nylon mesh, treated with RNase A and then stained with propidium iodide (50 mg L−1), which was dissolved in Otto’s buffer II (Otto et al., 1981). The C values were calculated according to the formula: 1Cobject = (G1 mean peak positionobject/G1 mean peak positionstandard) × 2C valuestandard.
The investigated species with their mating system, chromosome number, presence of ITRs, number (#) and distribution of 5S and 35S rDNA loci, rDNA organization (L, linked; S, separate; LS, mixed) and genome sizes. New chromosome numbers are shown in bold, with an asterisk indicating the presence of a small/mini chromosome. The infinity symbol (∞) indicates numerous FISH signals on all chromosomes, with numbers in parentheses indicating the minimum number of signals. A C-value for S. aequiloba could not be obtained because there was too little material. For authors of species names and voucher information see Supplementary Data Table S1
Species, sex . | n . | ITR . | # . | 5S rDNA . | # . | 35S rDNA . | rDNA . | 1C/pg . |
---|---|---|---|---|---|---|---|---|
Aneura pinguis Dioicous, female | 10 | 2 | 2 | Interstitial | 2 | Terminal | S | 7.7239 |
Chiloscyphus pallescens Monoicous | – | – | – | – | – | – | ? | 0.8353 |
Conocephalum conicum Dioicous, male | 9* | – | ∞ | Ter./Sub./Int. | ∞ | Ter./Sub./Int. | LS | 0.6776 |
Conocephalum conicum Dioicous, female | 9* | – | ∞ | Ter./Sub./Int. | ∞ | Ter./Sub./Int. | LS | 0.7698 |
Frullania dilatata Dioicous, male | 8 | – | 2 | Ter./Sub. | 3 | Ter./Sub. | LS | 0.5525 |
Frullania dilatata Dioicous, female | 9 | – | ∞ (8) | Ter./Sub./Int. | ∞ (8) | Ter./Sub./Int. | LS | 0.7607 |
Lejeunea cavifolia Monoicous | 18 | – | – | – | – | – | ? | 0.2203 |
Lunularia cruciata Dioicous, sterile | 9* | – | ∞ (7) | Ter./Sub./Int. | 2 | Terminal | S | 0.6893 |
Mesoptychia badensis Dioicous, sterile | 9* | – | – | – | – | – | ? | 0.5125 |
Pellia endiviifolia Dioicous, female | - | – | – | – | – | – | ? | 3.4942 |
Pellia endiviifolia Dioicous, male | 9 | – | ∞ | Ter./Sub./Int. | 2 | Terminal | S | 3.4081 |
Plagiochila asplenioides Dioicous, female | 9* | 1 | ∞ (6) | Ter./Sub./Int. | ? | ? | ? | 1.6919 |
Plagiochila asplenioides Dioicous, male | 9* | 3 | ∞ | Ter./Sub./Int. | ∞ | Ter./Sub./Int. | LS | 1.5358 |
Porella platyphylla Dioicous, male | 8 | – | ∞ | Ter./Sub./Int. | ∞ | Ter./Sub./Int. | LS | 1.0820 |
Radula complanata Monoicous | 15 | 2 | ∞ (13) | Ter./Sub./Int. | ∞ (13) | Ter./Sub./Int. | LS | 00.7370 |
Scapania aequiloba Dioicous, female | 8 | – | ∞ (6) | Ter./Sub./Int. | 1 | Terminal | S | – |
Species, sex . | n . | ITR . | # . | 5S rDNA . | # . | 35S rDNA . | rDNA . | 1C/pg . |
---|---|---|---|---|---|---|---|---|
Aneura pinguis Dioicous, female | 10 | 2 | 2 | Interstitial | 2 | Terminal | S | 7.7239 |
Chiloscyphus pallescens Monoicous | – | – | – | – | – | – | ? | 0.8353 |
Conocephalum conicum Dioicous, male | 9* | – | ∞ | Ter./Sub./Int. | ∞ | Ter./Sub./Int. | LS | 0.6776 |
Conocephalum conicum Dioicous, female | 9* | – | ∞ | Ter./Sub./Int. | ∞ | Ter./Sub./Int. | LS | 0.7698 |
Frullania dilatata Dioicous, male | 8 | – | 2 | Ter./Sub. | 3 | Ter./Sub. | LS | 0.5525 |
Frullania dilatata Dioicous, female | 9 | – | ∞ (8) | Ter./Sub./Int. | ∞ (8) | Ter./Sub./Int. | LS | 0.7607 |
Lejeunea cavifolia Monoicous | 18 | – | – | – | – | – | ? | 0.2203 |
Lunularia cruciata Dioicous, sterile | 9* | – | ∞ (7) | Ter./Sub./Int. | 2 | Terminal | S | 0.6893 |
Mesoptychia badensis Dioicous, sterile | 9* | – | – | – | – | – | ? | 0.5125 |
Pellia endiviifolia Dioicous, female | - | – | – | – | – | – | ? | 3.4942 |
Pellia endiviifolia Dioicous, male | 9 | – | ∞ | Ter./Sub./Int. | 2 | Terminal | S | 3.4081 |
Plagiochila asplenioides Dioicous, female | 9* | 1 | ∞ (6) | Ter./Sub./Int. | ? | ? | ? | 1.6919 |
Plagiochila asplenioides Dioicous, male | 9* | 3 | ∞ | Ter./Sub./Int. | ∞ | Ter./Sub./Int. | LS | 1.5358 |
Porella platyphylla Dioicous, male | 8 | – | ∞ | Ter./Sub./Int. | ∞ | Ter./Sub./Int. | LS | 1.0820 |
Radula complanata Monoicous | 15 | 2 | ∞ (13) | Ter./Sub./Int. | ∞ (13) | Ter./Sub./Int. | LS | 00.7370 |
Scapania aequiloba Dioicous, female | 8 | – | ∞ (6) | Ter./Sub./Int. | 1 | Terminal | S | – |
● Ter., Terminal; Sub., Subterminal; Int., Interstitial.
The investigated species with their mating system, chromosome number, presence of ITRs, number (#) and distribution of 5S and 35S rDNA loci, rDNA organization (L, linked; S, separate; LS, mixed) and genome sizes. New chromosome numbers are shown in bold, with an asterisk indicating the presence of a small/mini chromosome. The infinity symbol (∞) indicates numerous FISH signals on all chromosomes, with numbers in parentheses indicating the minimum number of signals. A C-value for S. aequiloba could not be obtained because there was too little material. For authors of species names and voucher information see Supplementary Data Table S1
Species, sex . | n . | ITR . | # . | 5S rDNA . | # . | 35S rDNA . | rDNA . | 1C/pg . |
---|---|---|---|---|---|---|---|---|
Aneura pinguis Dioicous, female | 10 | 2 | 2 | Interstitial | 2 | Terminal | S | 7.7239 |
Chiloscyphus pallescens Monoicous | – | – | – | – | – | – | ? | 0.8353 |
Conocephalum conicum Dioicous, male | 9* | – | ∞ | Ter./Sub./Int. | ∞ | Ter./Sub./Int. | LS | 0.6776 |
Conocephalum conicum Dioicous, female | 9* | – | ∞ | Ter./Sub./Int. | ∞ | Ter./Sub./Int. | LS | 0.7698 |
Frullania dilatata Dioicous, male | 8 | – | 2 | Ter./Sub. | 3 | Ter./Sub. | LS | 0.5525 |
Frullania dilatata Dioicous, female | 9 | – | ∞ (8) | Ter./Sub./Int. | ∞ (8) | Ter./Sub./Int. | LS | 0.7607 |
Lejeunea cavifolia Monoicous | 18 | – | – | – | – | – | ? | 0.2203 |
Lunularia cruciata Dioicous, sterile | 9* | – | ∞ (7) | Ter./Sub./Int. | 2 | Terminal | S | 0.6893 |
Mesoptychia badensis Dioicous, sterile | 9* | – | – | – | – | – | ? | 0.5125 |
Pellia endiviifolia Dioicous, female | - | – | – | – | – | – | ? | 3.4942 |
Pellia endiviifolia Dioicous, male | 9 | – | ∞ | Ter./Sub./Int. | 2 | Terminal | S | 3.4081 |
Plagiochila asplenioides Dioicous, female | 9* | 1 | ∞ (6) | Ter./Sub./Int. | ? | ? | ? | 1.6919 |
Plagiochila asplenioides Dioicous, male | 9* | 3 | ∞ | Ter./Sub./Int. | ∞ | Ter./Sub./Int. | LS | 1.5358 |
Porella platyphylla Dioicous, male | 8 | – | ∞ | Ter./Sub./Int. | ∞ | Ter./Sub./Int. | LS | 1.0820 |
Radula complanata Monoicous | 15 | 2 | ∞ (13) | Ter./Sub./Int. | ∞ (13) | Ter./Sub./Int. | LS | 00.7370 |
Scapania aequiloba Dioicous, female | 8 | – | ∞ (6) | Ter./Sub./Int. | 1 | Terminal | S | – |
Species, sex . | n . | ITR . | # . | 5S rDNA . | # . | 35S rDNA . | rDNA . | 1C/pg . |
---|---|---|---|---|---|---|---|---|
Aneura pinguis Dioicous, female | 10 | 2 | 2 | Interstitial | 2 | Terminal | S | 7.7239 |
Chiloscyphus pallescens Monoicous | – | – | – | – | – | – | ? | 0.8353 |
Conocephalum conicum Dioicous, male | 9* | – | ∞ | Ter./Sub./Int. | ∞ | Ter./Sub./Int. | LS | 0.6776 |
Conocephalum conicum Dioicous, female | 9* | – | ∞ | Ter./Sub./Int. | ∞ | Ter./Sub./Int. | LS | 0.7698 |
Frullania dilatata Dioicous, male | 8 | – | 2 | Ter./Sub. | 3 | Ter./Sub. | LS | 0.5525 |
Frullania dilatata Dioicous, female | 9 | – | ∞ (8) | Ter./Sub./Int. | ∞ (8) | Ter./Sub./Int. | LS | 0.7607 |
Lejeunea cavifolia Monoicous | 18 | – | – | – | – | – | ? | 0.2203 |
Lunularia cruciata Dioicous, sterile | 9* | – | ∞ (7) | Ter./Sub./Int. | 2 | Terminal | S | 0.6893 |
Mesoptychia badensis Dioicous, sterile | 9* | – | – | – | – | – | ? | 0.5125 |
Pellia endiviifolia Dioicous, female | - | – | – | – | – | – | ? | 3.4942 |
Pellia endiviifolia Dioicous, male | 9 | – | ∞ | Ter./Sub./Int. | 2 | Terminal | S | 3.4081 |
Plagiochila asplenioides Dioicous, female | 9* | 1 | ∞ (6) | Ter./Sub./Int. | ? | ? | ? | 1.6919 |
Plagiochila asplenioides Dioicous, male | 9* | 3 | ∞ | Ter./Sub./Int. | ∞ | Ter./Sub./Int. | LS | 1.5358 |
Porella platyphylla Dioicous, male | 8 | – | ∞ | Ter./Sub./Int. | ∞ | Ter./Sub./Int. | LS | 1.0820 |
Radula complanata Monoicous | 15 | 2 | ∞ (13) | Ter./Sub./Int. | ∞ (13) | Ter./Sub./Int. | LS | 00.7370 |
Scapania aequiloba Dioicous, female | 8 | – | ∞ (6) | Ter./Sub./Int. | 1 | Terminal | S | – |
● Ter., Terminal; Sub., Subterminal; Int., Interstitial.
RESULTS
Occurrence of L-type and S-type rDNA organization in liverworts
In total, we studied the cytotypes of nine dioicous and three monoicous species, although for some species we could not obtain both FISH data and C-values (Table 1). Our comparative approach required placing the species in a phylogenetic context, and we therefore added new sequences of the study species to the DNA matrix of Laenen et al. (2014), which resulted in a phylogeny with at least one species from 287 of the 386 currently recognized genera (Supplementary Data Fig. S1). The 12 species were placed in the phylogeny as expected from earlier studies.
Linked as well as separate 5S and 35S rDNA arrays were found in Concocephalum conicum, Porella platyphylla, Radula complanata, Frullania dilatata and Plagiochila asplenioides (Figs 1, 2B and 3C). Separate 5S and 35S rDNA loci (located on different chromosomes or far apart on the same chromosome) were found in Aneura pinguis, Lunularia cruciata, Pellia endiviifolia, and Scapania aequiloba (Table 1; Figs 2A, C and 3A, B).
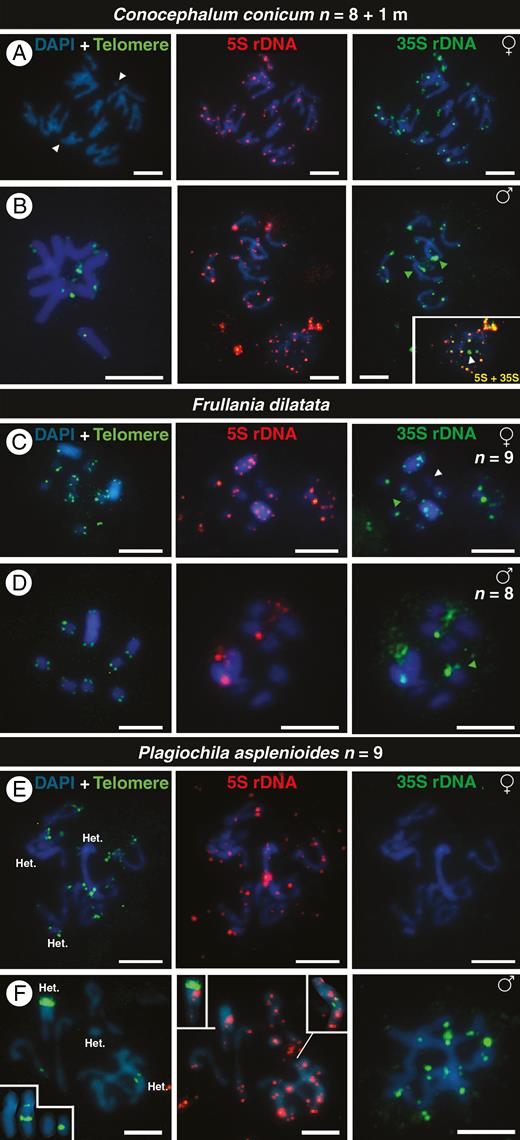
FISH signals of telomeres (green), 5S DNA (red) and 35S rDNA (green) in chromosomes of male and female individuals of the dioicous liverworts Conocephalum conicum (A, B), Frullania dilatata (C, D) and Plagiochila asplenioides (E, F). White arrowheads indicate small chromosomes or chromosomes without rDNA signals. Green arrowheads indicate the position of an isolated 35S rDNA signal. The inset in (B) shows the overlap of 5S and 35S rDNA signals in a nucleus in which a 35S locus is not linked with the 5S rDNA, and the insets in (F) show chromosomes with interstitial telomere repeats (ITRs). ‘Het.’ in (E) and (F) refers to heterochromatic chromosomes. The sex of each individual is given in the upper right of each row of metaphases. All chromosomes are counterstained with DAPI (blue). Scale bars = 5 μm.
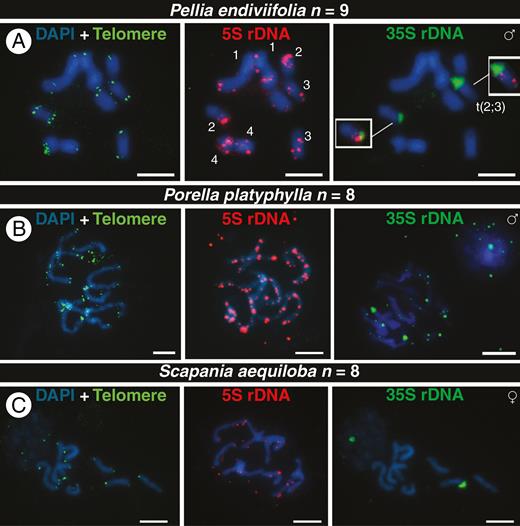
FISH signals of telomeres (green), 5S DNA (red) and 35S rDNA (green) in chromosomes of male and female individuals of the dioicous liverworts Pellia endiviifolia (A), Porella platyphylla (B) and Scapania aequiloba (C). Numbers in (C) show chromosome pairs based on the distribution of 5S rDNA signals, and the insets highlight a translocation among chromosomes 2 and 3 involving a 35S locus. The sex of each individual is given in the upper right of each row of metaphases. All chromosomes are counterstained with DAPI (blue). Scale bars = 5 μm.
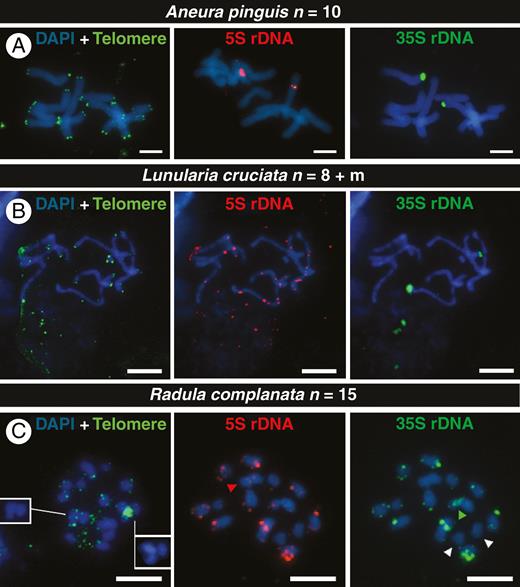
FISH signals of telomeres (green), 5S DNA (red) and 35S rDNA (green) signals in chromosomes of the monoicous liverworts Aneura pinguis (A), Lunularia cruciata (B) and Radula complanata (C). White arrowheads indicate chromosomes without rDNA signals, red ones show chromosome with only 5S rDNA (S-type), and green ones indicate the position of an isolated 35S rDNA signal. The insets in (C) show chromosomes with ITRs without the overlap with the telomere probe. All chromosomes are counterstained with DAPI (blue). Scale bars = 5 μm.
In the dioicous Conocephalum conicum, both sexes had n = 9 (8 + 1m; Fig. 1A, B) and telomere sequences were detected only after using high probe concentrations (Fig. 1B and Supplementary Data Fig. S2). In early mitotic anaphase (V shape) the chromosomes moved to the poles of the cell, suggesting the presence of monocentric centromeres, although we did not see them (Fig. 1A). All chromosomes of C. conicum showed rDNA signals (Fig. 1A, B), but most signals were small and distributed along chromosomes. Most of the 5S and 35S loci seemed to be of the L type (linked, see Introduction; Supplementary Data Fig. S3 shows the overlap between 5S and 35S), with the exception of distended 35S rDNA regions that seemed to be of the S type (separated; Fig. 1B inset and arrowheads; Supplementary Data Fig. S3). The mini chromosome in females possessed rDNA sequences, a feature absent in males.
In the likewise dioicous species Frullania dilatata, females had two large sex chromosomes (n = 7 + UU) and males a single large one (n = 7 + V). The sex chromosomes were heterochromatic and rich in AT sequences, which are preferentially stained by DAPI (Fig. 1C, D). There was a huge difference in the number of rDNA signals between the sexes. In female gametophytes, the two UU chromosomes presented 5S and 35S rDNA loci at subterminal and interstitial regions, similar to other chromosomes. The 5S and 35S rDNA signals were linked (L-type organization), with the exception of one chromosome having only 35S rDNA signal in one chromosome arm and another without any rDNA signals (Fig. 1C green and white arrowheads, respectively). In males, we detected two 5S rDNA signals, one in the terminal position of an autosome and another on the V chromosome. There were also three 35S rDNA signals, of which two were linked with the 5S locus and the third was located on the terminal region of an autosome (Fig. 1D). The number of rDNA signals differed among the cells of a single slide, with some chromosomes carrying only 35S rDNA and the two U chromosomes in some female cells differing in their rDNA signals (data not shown).
In dioicous Plagiochila asplenioides, male and female gametophytes both had n = 9, with two large chromosomes and one small chromosome that were heterochromatic (‘Het.’ in Fig. 1E, F). Interstitial telomere-like repeats were detected in the large heterochromatic chromosome in females (Fig. 1E and Table 1) as well as in the large and mid-sized chromosomes in males (Fig. 1F and inset). The sexes also differed in the number of their rDNA signals (compare Fig. 1E, F and insets). Similar to C. conicum and F. dilatata, most rDNA loci were of the L type, with the exception of a locus of 35S rDNA (Supplementary Data Fig. S4, arrowhead).
For the dioicous Porella platyphylla and Scapania aequiloba, both with n = 8, we managed to grow fertile plants only of one sex. The karyotype of P. platyphylla was rich in 5S rDNA repeats, with blocks of FISH signals along all chromosomes (Fig. 2B). With exception of one 35S locus, most 5S and 35S rDNA loci were linked, and the intensity of the former was higher than that of the latter (Supplementary Data Fig. S5). The karyotype of S. aequiloba exhibited weak interstitial signals of 5S and a single 35S rDNA locus located on different chromosomes, and hence is of the S type (Fig. 2C).
For the dioicous Pellia endiviifolia, we managed to grow both sexes, but obtained FISH preparations for the male sex only (Fig. 2A). The 5S rDNA signals allowed us to identify all chromosome pairs and revealed the move/translocation of a 35S rDNA locus from the terminal position of chromosome 2 to chromosome 3 (Fig. 2A). Consequently, one chromosome carried adjacent 5S and 35S rDNA signals while another carried both rDNA signals on the same chromosome arm, though at different positions (S type).
For the dioicous Aneura pinguis and Lunularia cruciata, we investigated meristems of young thalli. Aneura pinguis (Fig. 3A) had a chromosome number of n = 10, with two weak ITRs in a large chromosome (Supplementary Data Fig. S6). All other chromosomes had telomere FISH signals in the expected terminal positions. There were two 5S and 35S rDNA signals on different chromosomes (S type) at interstitial and terminal positions, respectively (Fig. 3A). Lunularia cruciata, with n = 8 + 1m, also presented large chromosomes with weak 5S rDNA signals interstitially located on most chromosomes, but only two 35S rDNA loci (Fig. 3B). One of the two chromosomes with a 35S rDNA locus, at a different chromosomal position, also had 5S rDNA loci (Fig. 3B).
Our telomere labelling helped us to determine the chromosome number, n = 15, of the monoicous species Radula complanata (Fig. 3C). Although its chromosomes were relatively small, we detected two ITRs on different chromosomes (Fig. 3C and Supplementary Data Fig. S7), one large and one small. The 5S and 35S rDNAs were co-localized (L type), with the exception of a distended 35S locus (green arrowhead in Fig. 3C), one chromosome with a single weak locus of 5S rDNA (red arrowhead in Fig. 3C), and two chromosomes without signals for both gene regions (white arrowheads in Fig. 3C).
Liverwort chromosome numbers and centromere types
Plotting of chromosome numbers for the clades of interest revealed that n = 8 and 9 were the most widespread numbers, with duplications (for instance n = 18) relatively rare (Supplementary Data Fig. S1). The two new chromosome numbers reported here, that of Lejeunea cavifolia (n = 18; species monoicous) and Scapania aequiloba (n = 8 in a female plant; Fig. 2C and Supplementary Data Fig. S8), confirm this general pattern. Morphologically, all studied chromosomes resembled holokinetic chromosomes in lacking centromere constrictions (Figs 1–3 and Supplementary Data Figs S2–S9).
Differences between male and female karyotypes and genome sizes, and presence of ITRs
Small or mini chromosomes were found in the dioicous Mesoptychia badensis (n = 9, 8 + 1m; Supplementary Data Fig. S8) and heteromorphic sex chromosomes in Frullania dilatata (Fig. 1C, D). The remaining dioicous species had large or small heterochromatic chromosomes, but always in both sexes, not just one sex. In the dioicous species, male genomes were smaller than female genomes, with differences ranging from 0.0861 pg per 1C in Pellia endiviifolia, 0.0922 pg per 1C in Conocephalum conicum and 0.1561 pg per 1C in Plagiochila asplenioides to 0.2082 pg per 1C in Frullania dilatata, the last being attributable to the presence of two large U chromosomes in females. Overall, genome sizes varied from 0.2203 pg per 1C in Lejeunea cavifolia to 7.7239 pg per 1C in Aneura pinguis (Table 1), and the species with the largest C values also had the largest chromosomes: (in increasing order) Porella platyphylla, Pellia endiviifolia and Aneura pinguis (Figs 2A, B and 3A).
DISCUSSION
Occurrence of L-type, S-type, and L-S-type organization of rDNA arrays in liverworts
The 18S, 5.8S and 35S rRNA genes and the 5S rRNA genes of land plants are either located apart from each other (separate or S type) or linked (L type), such that 5S rRNA is inserted within the 35S repeat unit (Roa and Guerra, 2012, 2015; Garcia et al., 2014, 2017). The most comprehensive study so far analysed 2949 karyotypes from 1791 species in 86 families, with 87 % having S-type organization, causing the authors to suggest that S-type organization might be the ancestral condition in land plants (Garcia et al., 2017). This analysis, however, included only three ferns, two bryophytes and no algae (the outgroup).
In liverworts, the S-type rDNA organization is present in four of the nine species studied here (Figs 2A, C and 3A, B; Aneura pinguis, Lunularia cruciata, Scapania aequiloba, Pellia endiviifolia) and in two of four Pellia species studied earlier (Orzechowska et al., 2010, 2018). In the phylogeny, species with S-type rDNA are placed close to species with L-S-type rDNA (Supplementary Data Fig. S1), implying evolutionary switches (next section). For example, within Marchantiopsida, Lunularia presents the S type while the genus Conocephalum has the L-S rDNA type, and in Jungermanniopsida only Scapania aequiloba exhibited the S-type arrangement. In angiosperms, a mixed L-S rDNA arrangement has been reported from ten species of subfamily Aurantioideae (Barros e Silva et al., 2013; their Fig. 3), five species of Altroemeria (Baeza et al., 2007; Chacón et al., 2012), ten species and subspecies of Brassica (Hasterok et al., 2006) and three species of Avena (Shelukhina et al., 2007). In gymnosperms, this type of rDNA organization is unknown (Garcia and Kovařík, 2013; their Table 1).
Evolutionary lability of rDNA arrays within the small genus Pellia
In the genus Pellia, 5S and 35S rDNA signals have now been studied in four of its six species. Three have n = 9, but P. borealis with n = 18 appears to be an allotetraploid (Orzechowska et al., 2010). Pellia epiphylla has four 5S rDNA sites, of which one is adjacent to the single 35S rDNA of the karyotype; P. borealis has five 5S rDNA sites, one linked with one of the two 35S rDNA signals (Orzechowska et al., 2010); P. endiviifolia males have three 5S rDNA sites, two of them linked with the two 35S rDNA in the FISH images of Orzechowska et al. (2010), and P. neesiana females have two 5S rDNA sites and four of 35S rDNA of the S type (Orzechowska et al., 2010, 2018). With the 5S rDNA signals as chromosome markers, we could identify all chromosome pairs of a P. endiviifolia male and, using this karyotype as a reference, we inferred that the S-type locus of 35S rDNA moved/jumped from chromosome 2 to 3 (Fig. 2A inset). A similar jump is seen in a P. neesiana female, although Orzechowska et al. (2010) did not comment on this.
Few sex-specific differences in rDNA arrays and absence of interstitial telomere repeat (ITR) signals in sex chromosomes
We detected differences in the number of rDNA loci between the two sexes in Plagiochila asplenioides (Fig. 1E, F: different 5S rDNA signals in males and in females) and in Frullania dilatata (Fig. 1C, D), which has two U chromosomes with three signals each of L-type rDNA (Fig. 1C) and a single V chromosome with only one such signal (Fig. 1D). The C-value measurements illustrate the ‘weight’ of the U chromosomes (Table 1; Fig. 1C). The U chromosome of M. polymorpha also has L-type rDNA (Nakayama et al., 2001; Fujisawa et al., 2003), estimated to account for 12–14 Mb of the 280 Mb of its genome size (Okada et al., 2000; male/female differences in C values have not been determined). Sex differences in rDNA patters in M. polymorpha, however, are small (Nakayama et al., 2001; Fujisawa et al., 2003), and in Conocephalum conicum, studied here, they are restricted to a mini chromosome (Fig. 1A, B and Supplementary Data Fig. S3). In the latter species we observed in meiosis I that homologous paternal and maternal chromosomes pair side by side with the exception of regions where one chromosome of each pair has a large 5S rDNA signal while its homologous chromosome does not (Supplementary Data Fig. S9).
The ITRs detected in Aneura pinguis and Plagiochila asplenioides (Table 1) were not restricted to any obvious sex chromosomes. For example, the female karyotype of P. asplenioides had a single ITR signal on the middle of the largest heterochromatic chromosome (Fig. 1E), and the male karyotype had two ITRs (one large and one small) and additionally a large signal in another heterochromatic chromosome (Fig. 1F), which does not suggest sex chromosome-specific ITRs. The ITRs in Radula complanata, a monoicous species, however, revealed recent chromosomal rearrangements, which could have occurred after a polyploidization event since plants from Bavaria had n = 15, but plants from France and other localities in Germany have n = 8 (Fritsch, 1991). All telomeric repeats were Arabidopsis-like.
Given these results, it remains unclear whether Bull’s (1978, 1983) expectation of bryophyte male and female sex chromosomes having identical evolutionary trajectories will hold across liverworts.
Conserved chromosome numbers across liverworts
Chromosome numbers are available for a small fraction of the ~7000 species of liverworts, and sexual systems also are insufficiently known. So far, chromosome numbers of n = 8 or 9 dominate, with the exception of a few genera with larger numbers, usually multiples of 9, such as 18 and 36 (Supplementary Data Fig. S1). The occurrence of transitions from 7 to 14, 8 to 16, 9 to 18 and 10 to 20 can also be seen in Supplementary Data Fig. S1. The low chromosome numbers do not consistently correlated with low C values, although most C values are below 1 pg per 1C (Temsch et al., 2010; Bainard et al., 2013; our study, Table 1). Based on the current sampling, the genera with high variability of chromosome numbers are Asterella and Riccia (highlighted section 1 of Supplementary Data Fig. S1), Scapania (section 4 of Supplementary Data Fig. S1) and Plagiochila (section 6 of Supplementary Data Fig. S1). We determined that the dioicous Scapania aequiloba has n = 8 chromosomes (in females) and the monoicous Lejeunea cavifolia n = 18, while Fritsch (1991) gives n = 8 + 1 and 10 for the former and n = 10 for the latter.
Lack of distinctness of centromeres and chromosome behaviour in mitosis
Liverwort chromosomes lack a clear primary constriction, instead resembling holokinetic chromosomes (Sousa et al., 2011). Nevertheless, the migration of liverwort chromosomes in mitotic anaphase suggests that they are monocentric chromosomes (Heitz, 1928; Lorbeer, 1934; Tinney, 1935; our Fig. 1A). As far as is known, bryophyte nuclei have well-defined microtubule-organizing centres (MTOCs) comparable to the centrosomes of animals (Brown and Lemmon, 1990, 2004; Shimamura et al., 2004). This may explain why centromeres in bryophyte chromosomes have a different organization from those of vascular plants.
Conclusions
The two types of rDNA rearrangement (S and L) in liverworts appear to have evolved repeatedly, and the occurrence of linked and non-linked rDNA in the same species seems common (based on the few species so far investigated). The evidence for differential accumulation of rDNA between the sexes is limited, perhaps because in haploid-dominant organisms there is no heterogametic and homogametic sex (Bull, 1978, 1983), and instead either the U or the V chromosome may idiosyncratically accumulate repetitive DNA.
SUPPLEMENTARY DATA
Supplementary data are available online at https://dbpia.nl.go.kr/aob and consist of the following. Table S1: voucher information and GenBank accession numbers of studied species included in the DNA matrix of Laenen et al. (2014). Figure S1: maximum likelihood tree for 317 species from 287 of 386 currently recognized liverwort genera. Figure S2: telomere detection in a Conocephalum conicum female. Figure S3: overlap of 5S and 35S rDNA signals to verify the linkage of these gene regions in a Conocephalum conicum female. Figure S4: detection of 5S and 35S rDNA signals in a Plagiochila asplenioides male. Figure S5: detection of 5S and 35S rDNA signals in a Porella platyphylla male. Figure S6: detection of ITRs in Aneura pinguis. Figure S7: detection of ITRs in Radula complanata. Figure S8: chromosome counts for Mesoptychia badensis and Lejeunea cavifolia. Figure S9: detection of 5S rDNA signals in meiosis I of Conocephalum conicum.
ACKNOWLEDGEMENTS
We thank Cymon Cox and an anonymous reviewer for insightful comments, Professor C. Oberprieler, Institute of Plant Sciences, University of Regensburg, for permission to use his facilities, and A. Metko and Dr M. Silber for help with laboratory work.
FUNDING
Financial support for this project came from the Deutsche Forschungsgemeinschaft grant HE 3584/6-1, which supported J.B.