-
PDF
- Split View
-
Views
-
Cite
Cite
Gunnar Keppel, Gianluigi Ottaviani, Susan Harrison, Grant W Wardell-Johnson, Matteo Marcantonio, Ladislav Mucina, Towards an eco-evolutionary understanding of endemism hotspots and refugia, Annals of Botany, Volume 122, Issue 6, 2 November 2018, Pages 927–934, https://doi.org/10.1093/aob/mcy173
- Share Icon Share
Abstract
Refugia are island-like habitats that are linked to long-term environmental stability and, as a result, high endemism. Conservation of refugia and endemism hotspots should be based on a deep ecological and evolutionary understanding of their functioning, which remains limited. Although functional traits can provide such insights, a corresponding, coherent framework is lacking.
Plant communities in refugia and endemism hotspots should, due to long-term environmental stability, display unique functional characteristics linked to distinct phylogenetic patterns. Therefore, such communities should be characterized by a functional signature that exhibits: (1) distinct values and combinations of traits, (2) higher functional diversity and (3) a prevalence of similar traits belonging to more distantly related lineages inside, compared to outside, of endemism hotspots and refugia. While the limited functional trait data available from refugia and endemism hotspots do not allow these predictions to be tested rigorously, three potential applications of the functional signature in biogeography and conservation planning are highlighted. Firstly, it allows the functional characteristics of endemism hotspots and refugia to be identified. Secondly, the strength of the functional signature can be compared among these entities, and with the surrounding landscape, to provide an estimate of the capacity of endemism hotspots and refugia to buffer environmental changes. Finally, the pattern of the functional signature can reveal ecological and evolutionary processes driving community assembly and functioning, which can assist in predicting the effect of environmental changes (e.g. climate, land-use) on communities in endemism hotspots and refugia.
The proposed functional signature concept allows the systematic integration of plant functional traits and phylogeny into the study of endemism hotspots and refugia, but more data on functional traits in these entities are urgently needed. Overcoming this limitation would facilitate rigorous testing of the proposed predictions for the functional signature, advancing the eco-evolutionary understanding of endemism hotspots and refugia.
INTRODUCTION
Refugia provide more stable environmental conditions (e.g. climate, resource availability) for biota than the surrounding landscape, either through large areas that are environmentally buffered (macrorefugia) or by environmental heterogeneity facilitating the retention of smaller areas with suitable habitats (microrefugia) within close proximity (Rull, 2009; Ashcroft, 2010; Dobrowski, 2011; Keppel et al., 2012; Costa et al., 2018). Refugia therefore affect past, current and future distributions of biota that can retreat to these more benign, island-like habitats during unfavourable conditions (Fig. 1), and then potentially recolonize the surrounding landscape when conditions again become favourable (Taberlet et al., 1998; Svenning et al., 2008). Hence, refugia can be considered spatially distinct geographical units (Ashcroft et al., 2009, 2012; Mackey et al., 2012) of relative long-term environmental stability (Tzedakis et al., 2002; Taberlet and Cheddadi, 2002; Médail and Diadema, 2009).
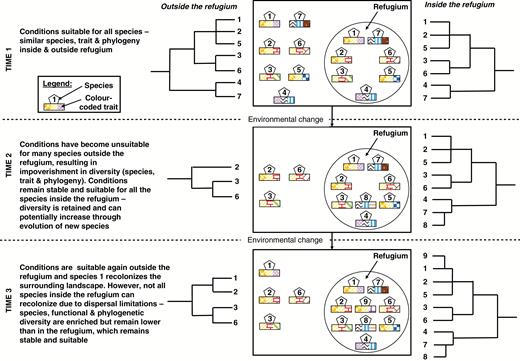
Schematic representation of the eco-evolutionary emergence of a refugium, indicating the traits and phylogeny of species inside and outside of a refugium at three time periods separated by environmental change. The number of species (indicated numbers), functional traits (colour-coded squares in rectangles) and phylogenetic diversity (illustrated by phylogenetic trees) are retained and increase inside refugia compared to the surrounding landscape, which is experiencing harsher environmental conditions.
The conditions of stability and isolation in refugia (when compared to similar habitats that are not refugia) promote the persistence and evolution of species, producing high endemism (Kier et al., 2009; Harrison and Noss, 2017; Sandel et al., 2017). Macrorefugia would be expected to support larger populations and more species and are hence considered to have a larger capacity to support biodiversity (Keppel et al., 2015). However, several microrefugia could also combine to provide a high-capacity refugium, provided there is sufficient connectivity. In addition to size, the amount of environmental stability and microclimatic heterogeneity have been proposed to influence the capacity of refugia (Keppel et al., 2015). Here we will focus on refugia with a high capacity to enable the persistence of biodiversity by providing relatively stable conditions over long periods of time, whether this capacity is facilitated by a macrorefugium or an aggregation of several microrefugia.
Such high-capacity refugia facilitate the persistence and evolution of species and are therefore strongly linked to endemism hotspots (Weber et al., 2014; Harrison and Noss, 2017; Molina-Venegas et al., 2017). Indeed, stable environments as offered by refugia are considered a major contributing formation to many of the 36 global biodiversity hotspots, each of which has >1500 endemic species (Myers et al., 2000; Harrison and Noss, 2017). Despite this link, the concept of a refugium is independently relevant for conservation planning, because refugia of lower capacity may still contribute to species survival during environmental change by being holdouts and stepping stones (Keppel and Wardell-Johnson, 2015), and because many past refugia may not remain stable under future anthropogenic climate change (Mokany et al., 2017). As a result, both endemism hotspots and refugia are key entities for biodiversity conservation under environmental change (Myers et al., 2000; Keppel and Wardell-Johnson, 2012; Morelli et al., 2016).
Effective conservation requires a good understanding of the ecological and evolutionary processes shaping biotic communities (Balmford and Cowling, 2006). Plant functional traits can provide such insights, especially when combined with phylogenetic analyses (Kooyman et al., 2011; Pavoine and Bonsall, 2011; Molina-Venegas et al., 2015). Functional trait studies have the potential to detect contemporary ecological mechanisms driving community assembly (Lavorel and Garnier, 2002; Violle et al., 2007; Götzenberger et al., 2012) and to inform on the ‘evolutionary heritage’ of current trait patterns (Molina-Venegas et al., 2015, 2017; de Bello et al., 2017).
However, few studies have investigated plant functional traits within endemism hotspots or refugia (see Table 1 for examples). Furthermore, the complexity of ecological and evolutionary processes often prevents clear predictions of trait patterns. For example, the same process (e.g. competition) may generate different patterns (both trait convergence and divergence), or the same trait pattern may be produced by different mechanisms (see de Bello et al., 2012 for details). In addition, various ecological processes operate simultaneously and at different scales, further complicating the detection of prevalent mechanisms (de Bello et al., 2012; Götzenberger et al., 2012; Spasojevic and Suding, 2012; Gross et al., 2013). Coupling trait data with phylogenetic analyses can help to disentangle the various eco-evolutionary processes determining trait expression (Molina-Venegas et al., 2015, 2017; Dayrell et al., 2017; de Bello et al., 2017).
Potential plant functional traits that should contribute to the unique functional signature of refugia in general, and to that of mesic (wetter) and pyric (lower fire frequency) refugia in particular. Refugia retain more stable environmental conditions, allowing on-spot persistence of plants over evolutionary time scales (e.g. Australian Wet Tropics, Brazilian campos rupestre). Note that this table provides examples and is not an exhaustive list
Trait . | Expectations for trait in refugium . | Example references . |
---|---|---|
Traits associated with generally more stable and favourable environmental conditions | ||
Lifespan | More long-lived plants due to stable conditions | Bhagwat and Willis (2008); Mosblech et al. (2011); De Smedt et al. (2018) |
Reproduction type | Prevalence of vegetative (less genetic variation) over riskier sexual reproduction | Dayrell et al. (2017); Jiménez-Alfaro et al. (2016) |
Seed dormancy | Low dormancy associated with lower investment in sexual reproduction | Dayrell et al. (2017) |
Seed size & mass | Large, heavy seeds due to less need for long- distance dispersal | Rossetto and Kooyman (2005); Bhagwat and Willis (2008); Kooyman et al. (2011); Weber et al. (2014) |
Traits associated with consistently cooler and moister (more mesic) conditions, as found on north-facing slopes in the Northern Hemisphere | ||
Plant height | Small plants associated with ecological limits imposed by cold, wind stress and high elevations | Doležal et al. (2012); Copeland and Harrison (2015) |
Specific leaf area (SLA) | High SLA as a result of reduced sclerophylly and more acquisitive nutrient uptake strategies | Spasojevic et al. (2013); Copeland and Harrison (2015) |
Dispersal mode | Low proportion of wind-dispersed species, related to more investments in on-spot persistence | Copeland and Harrison (2015) |
Traits associated with consistently lower fire frequency as found on some Australian rocky outcrops | ||
Fire response (seeders vs. resprouters) | Persistence of obligate-seeders (killed by fire) | Clarke (2002); Enright et al. (2014) |
Serotiny (seed storage in canopy and release after fire) | Prevalence of serotinous plants, a less resilient recruitment strategy to cope with changing fire regimes | Enright et al. (2014) |
Trait . | Expectations for trait in refugium . | Example references . |
---|---|---|
Traits associated with generally more stable and favourable environmental conditions | ||
Lifespan | More long-lived plants due to stable conditions | Bhagwat and Willis (2008); Mosblech et al. (2011); De Smedt et al. (2018) |
Reproduction type | Prevalence of vegetative (less genetic variation) over riskier sexual reproduction | Dayrell et al. (2017); Jiménez-Alfaro et al. (2016) |
Seed dormancy | Low dormancy associated with lower investment in sexual reproduction | Dayrell et al. (2017) |
Seed size & mass | Large, heavy seeds due to less need for long- distance dispersal | Rossetto and Kooyman (2005); Bhagwat and Willis (2008); Kooyman et al. (2011); Weber et al. (2014) |
Traits associated with consistently cooler and moister (more mesic) conditions, as found on north-facing slopes in the Northern Hemisphere | ||
Plant height | Small plants associated with ecological limits imposed by cold, wind stress and high elevations | Doležal et al. (2012); Copeland and Harrison (2015) |
Specific leaf area (SLA) | High SLA as a result of reduced sclerophylly and more acquisitive nutrient uptake strategies | Spasojevic et al. (2013); Copeland and Harrison (2015) |
Dispersal mode | Low proportion of wind-dispersed species, related to more investments in on-spot persistence | Copeland and Harrison (2015) |
Traits associated with consistently lower fire frequency as found on some Australian rocky outcrops | ||
Fire response (seeders vs. resprouters) | Persistence of obligate-seeders (killed by fire) | Clarke (2002); Enright et al. (2014) |
Serotiny (seed storage in canopy and release after fire) | Prevalence of serotinous plants, a less resilient recruitment strategy to cope with changing fire regimes | Enright et al. (2014) |
Potential plant functional traits that should contribute to the unique functional signature of refugia in general, and to that of mesic (wetter) and pyric (lower fire frequency) refugia in particular. Refugia retain more stable environmental conditions, allowing on-spot persistence of plants over evolutionary time scales (e.g. Australian Wet Tropics, Brazilian campos rupestre). Note that this table provides examples and is not an exhaustive list
Trait . | Expectations for trait in refugium . | Example references . |
---|---|---|
Traits associated with generally more stable and favourable environmental conditions | ||
Lifespan | More long-lived plants due to stable conditions | Bhagwat and Willis (2008); Mosblech et al. (2011); De Smedt et al. (2018) |
Reproduction type | Prevalence of vegetative (less genetic variation) over riskier sexual reproduction | Dayrell et al. (2017); Jiménez-Alfaro et al. (2016) |
Seed dormancy | Low dormancy associated with lower investment in sexual reproduction | Dayrell et al. (2017) |
Seed size & mass | Large, heavy seeds due to less need for long- distance dispersal | Rossetto and Kooyman (2005); Bhagwat and Willis (2008); Kooyman et al. (2011); Weber et al. (2014) |
Traits associated with consistently cooler and moister (more mesic) conditions, as found on north-facing slopes in the Northern Hemisphere | ||
Plant height | Small plants associated with ecological limits imposed by cold, wind stress and high elevations | Doležal et al. (2012); Copeland and Harrison (2015) |
Specific leaf area (SLA) | High SLA as a result of reduced sclerophylly and more acquisitive nutrient uptake strategies | Spasojevic et al. (2013); Copeland and Harrison (2015) |
Dispersal mode | Low proportion of wind-dispersed species, related to more investments in on-spot persistence | Copeland and Harrison (2015) |
Traits associated with consistently lower fire frequency as found on some Australian rocky outcrops | ||
Fire response (seeders vs. resprouters) | Persistence of obligate-seeders (killed by fire) | Clarke (2002); Enright et al. (2014) |
Serotiny (seed storage in canopy and release after fire) | Prevalence of serotinous plants, a less resilient recruitment strategy to cope with changing fire regimes | Enright et al. (2014) |
Trait . | Expectations for trait in refugium . | Example references . |
---|---|---|
Traits associated with generally more stable and favourable environmental conditions | ||
Lifespan | More long-lived plants due to stable conditions | Bhagwat and Willis (2008); Mosblech et al. (2011); De Smedt et al. (2018) |
Reproduction type | Prevalence of vegetative (less genetic variation) over riskier sexual reproduction | Dayrell et al. (2017); Jiménez-Alfaro et al. (2016) |
Seed dormancy | Low dormancy associated with lower investment in sexual reproduction | Dayrell et al. (2017) |
Seed size & mass | Large, heavy seeds due to less need for long- distance dispersal | Rossetto and Kooyman (2005); Bhagwat and Willis (2008); Kooyman et al. (2011); Weber et al. (2014) |
Traits associated with consistently cooler and moister (more mesic) conditions, as found on north-facing slopes in the Northern Hemisphere | ||
Plant height | Small plants associated with ecological limits imposed by cold, wind stress and high elevations | Doležal et al. (2012); Copeland and Harrison (2015) |
Specific leaf area (SLA) | High SLA as a result of reduced sclerophylly and more acquisitive nutrient uptake strategies | Spasojevic et al. (2013); Copeland and Harrison (2015) |
Dispersal mode | Low proportion of wind-dispersed species, related to more investments in on-spot persistence | Copeland and Harrison (2015) |
Traits associated with consistently lower fire frequency as found on some Australian rocky outcrops | ||
Fire response (seeders vs. resprouters) | Persistence of obligate-seeders (killed by fire) | Clarke (2002); Enright et al. (2014) |
Serotiny (seed storage in canopy and release after fire) | Prevalence of serotinous plants, a less resilient recruitment strategy to cope with changing fire regimes | Enright et al. (2014) |
Despite the current limitations, i.e. lack of trait data and challenges to infer processes from trait patterns, we argue that the inherent environmental stability of refugia should create unique combinations of traits that could provide important insights about eco-evolutionary processes at the community level, especially when combined with phylogenetic data. Endemism hotspots should also be associated with these unique functional and phylogenetic characteristics, due to the aforementioned contribution of refugia to their formation. It has been argued that a whole-community, trait-based approach is urgently needed and could make valuable contributions to understanding evolution and ecology in endemism hotspots and refugia (Hampe et al., 2013; Harrison and Noss, 2017). We therefore propose a coherent conceptual framework to investigate the unique functional characteristics of these entities through systematic integration of functional and phylogenetic analyses.
We propose that the consistent, long-term, eco-evolutionary selective forces in endemism hotspots and refugia produce a functional signature potentially characterized by (1) distinct values and combinations of traits, (2) higher functional diversity (FD) and (3) functionally similar traits belonging to distantly related lineages. We argue that implementation of the proposed framework will produce essential insights about the eco-evolutionary functioning of endemism hotspots and refugia. Furthermore, we highlight three important applications of the functional signature: (1) description of the functional composition, (2) comparison of functional characteristics, and (3) inference of ecological and evolutionary drivers of community assembly in endemism hotspots and refugia, especially when compared with the surrounding landscape (Fig. 2). Such knowledge could be applied to predicting likely past and future responses to environmental changes (e.g. climate, land-use) of plant species and communities in endemism hotspots and refugia. However, as a first step, an understanding of the spatial, temporal and environmental context for the endemism hotspots or refugia under consideration is needed.
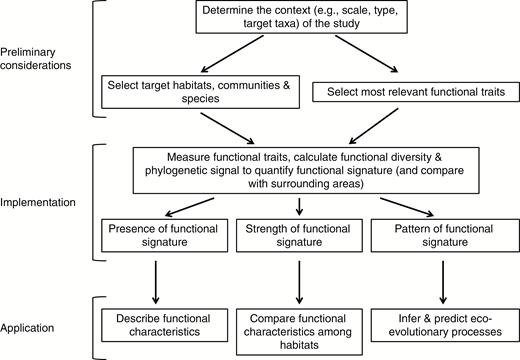
A conceptual framework for integrating functional traits in research on endemism hotspots and refugia using the functional signature.
CLARIFYING THE CONTEXT
Spatial and temporal parameters define endemism hotspots and refugia (Myers et al., 2000; Bennett and Provan, 2008; Keppel and Wardell-Johnson, 2015). The location of these habitats may differ among taxonomic groups (Stewart et al., 2010; Mosblech et al., 2011; Howard et al., 2015). For example, while the Southwest Pacific Islands are a hotspot for plant endemism (Keppel et al., 2016; Ibanez et al., 2018), this is not the case for mammals (Flannery, 1995). Furthermore, a refugium for cold-adapted species may not be one for species adapted to warmer conditions (Bátori et al., 2017; Mokany et al., 2017).
The spatial grain used in a study of endemism hotspots and refugia is of central importance and needs to be appropriate for the target organism, as demonstrated in the identification of suitable future habitats in the Californian Floristic Province (Ackerly et al., 2010; Franklin et al., 2013). In addition, endemism hotspots and refugia can differ considerably in geographical size. For example, refugia can range from large areas facilitating the persistence of entire remnant ecosystems (i.e. macrorefugia; Kooyman et al., 2011; Dayrell et al., 2017; Molina-Venegas et al., 2017) to unique microhabitats supporting specific taxa and local biotic communities (i.e. microrefugia; Rull, 2009; Stewart et al., 2010; Mosblech et al., 2011).
Time is another relevant factor when considering the dynamics of endemism hotspots and refugia (Keppel et al., 2012). For example, endemism hotspots may be of varying age, affecting the proportion of palaeo- and neoendemic species (Kraft et al., 2010; Molina-Venegas et al., 2017). Furthermore, refugia are known to fluctuate through time with regard to size and prevalent environmental conditions (Graham et al., 2010; Keppel and Wardell-Johnson, 2015; Mokany et al., 2017). Some refugia are indeed transient, providing environmental conditions that are unique from the surrounding landscape only for a limited period (Graham et al., 2010; Keppel and Wardell-Johnson, 2015).
Finally, it is key to consider the key environmental stressor(s) a refugium provides relief from, when compared to the surrounding landscape (Keppel et al., 2012; Reside et al., 2014; Table 1). For example, mesic refugia buffer against increasing aridification (Byrne, 2008; Mosblech et al., 2011; McLaughlin et al., 2017), thermal refugia provide relatively stable temperatures through time (Bhagwat and Willis, 2008; Stewart et al., 2010; Dobrowski, 2011) and pyric refugia have a lower frequency of fire (Clarke, 2002; Schwilk and Keeley, 2006; De Smedt et al., 2018).
THE FUNCTIONAL SIGNATURE
Biotic communities are shaped by ecological and evolutionary processes, such as species coexistence and filtering, according to the prevailing biotic interactions and environmental conditions (Fukami et al., 2005; de Bello et al., 2012; Spasojevic and Suding, 2012). The ecological and evolutionary histories of plant communities are reflected in the life histories and functional trait compositions of organisms (Weiher and Keddy, 1995; Díaz and Cabido, 1997). As a result, communities generally display unique sets of functional characteristics that relate to the major abiotic and biotic drivers and are manifested in trait values and functional metrics. These distinct trait values and functional metrics define the functional signature of a community (Hunt et al., 2004; Pakeman et al., 2009).
In the context of both endemism hotspots and refugia, functional traits and phylogeny should reflect the prevalent evolutionary and ecological processes associated with long-term stability and insularity (Kooyman et al., 2011; Molina-Venegas et al., 2015; Fig. 1). Phylogenies should reflect the long-term persistence of lineages, reflected in high palaeoendemism (Mishler et al., 2014; Molina-Venegas et al., 2017; Smyčka et al., 2017). Furthermore, the environmental stability of these entities imposes a cascade of abiotic and biotic trait filters at different spatial scales, as in any other ecosystem (de Bello et al., 2012; Götzenberger et al., 2012). This eco-evolutionary, continuous and consistent filtering process should select for species more distantly related phylogenetically and characterized by unique, highly conserved functional traits that are adapted to the stable environmental conditions inside the endemism hotspot or refugium (e.g. Dayrell et al., 2017; Molina-Venegas et al., 2017), compared to similar habitats in the less stable surrounding landscape (Costa et al., 2018; Fig. 1).
These unique and conserved functional traits should be reflected in the expression (values and range), combinations and FD of traits (Figs 1 and 2). Traits associated with poor dispersal, which should be favoured in environments with long-term stability, have indeed been found to be more prevalent in refugia (Bhagwat and Willis, 2008; Weber et al., 2014; Costa et al. 2018). Similarly, the campo rupestre grasslands in Brazil, which are considered an endemism hotspot and macrorefugium (Silveira et al., 2016; Mucina, 2018) that has experienced long-term stability, have a very high proportion of species with non-dormant seeds when compared with communities outside this endemism hotspot (Dayrell et al., 2017).
The long-term environmental stability of endemism hotspots and refugia should also influence FD patterns (Fig. 1). FD is defined as the extent of trait variability in a unit of study (Petchey and Gaston, 2002; Villéger et al., 2008; Laliberté and Legendre, 2010). Furthermore, environmental stability should facilitate strong biotic interactions (e.g. competition, facilitation within and among species), allowing for fine-tuning of plant–plant interactions at both habitat and micro-habitat scales (Kooyman et al., 2011; de Bello et al., 2012). These interactions, and continuously favourable environmental conditions, should facilitate the persistence of species and functional traits that are purged from the less stable surrounding landscape (Fig. 1). We suggest that endemism hotspots and refugia should therefore exhibit higher FD values (see Ordonez and Svenning, 2015). However, as mentioned earlier, trait patterns can be hard to predict and may not be linked to a single, prevalent ecological process (de Bello et al., 2012; Spasojevic and Suding, 2012; Gross et al., 2013). Therefore, FD may not always be higher inside endemism hotspots and refugia.
Functional redundancy (FRed) measures the degree to which similar traits are present in multiple species of an assemblage and indicates the degree to which organisms have evolved to carry out similar functional roles (Rosenfeld, 2002; Laliberté et al., 2010). While FRed would be expected to differ inside and outside of endemism hotspots and refugia, such patterns are challenging to predict, and no study, to our knowledge, has investigated this. For example, similar functional characteristics may be selected for in traits that are sensitive to stable environmental conditions, producing higher FRed values. Alternatively, stability may facilitate the persistence of traits that disappear outside endemism hotspots and refugia, reducing FRed values. Detailed studies will be required to determine the tendencies of FRed in refugia and endemism hotspots, and we therefore have not made concrete predictions here.
Traits in endemism hotspots and refugia should also be strongly linked to evolutionary history, due to the evolutionary constraints imposed by persistent stability and isolation. The evolutionary effect on functional traits can be investigated either by jointly examining separate functional and phylogenetic patterns (Kooyman et al., 2011; Molina-Venegas et al., 2015), by determining the variation in a trait explained by phylogeny (Desdevises et al., 2003) or by quantifying the phylogenetic signal in trait expression (Blomberg et al., 2003; Dayrell et al., 2017; Molina-Venegas and Rodríguez, 2017). A strong phylogenetic signal indicates co-occurring species that are more closely related and more functionally similar to each other than expected by chance (Blomberg et al., 2003, Molina-Venegas and Rodríguez, 2017). However, phylogenetic patterns of communities can be strongly influenced by dispersal and the evolutionary history of immigrants (Taylor and Keller, 2007; Wilson et al., 2009).
Endemism hotspots and refugia should therefore have a distinct functional signature (Figs 1 and 2). We hypothesize that biota in these entities should exhibit the following characteristics (when compared to the surrounding, less stable landscape of the same habitat type):
(1) different trait expression and unique suites of functional traits,
(2) greater FD, and
(3) functionally similar traits belonging to deeply related lineages in phylogenetic trees.
These hypotheses should be rigorously tested, as should patterns of FRed, to determine whether these are indeed consistent features of refugia. Whether or not these predictions are supported, results will provide essential insights into the characteristics and eco-evolutionary functioning of refugia and endemism hotspots.
USING THE FUNCTIONAL SIGNATURE
As mentioned above, specifying the context is key when studying endemism hotspots or refugia. It is a crucial first step that will facilitate selection of the most appropriate functional traits, i.e. those expected to be sensitive to key environmental stressors and ecosystem properties (Fig. 2). For example, seed size and seed mass are good candidate traits for quantifying the functional signature of refugia and endemism hotspots in the tropical and subtropical rain forests of eastern Australia, which experienced severe contractions during the glacial cycles (Rossetto and Kooyman, 2005; Kooyman et al., 2011; Weber et al., 2014; Table 1).
Once selected, functional traits can be measured; FD and the phylogenetic signal can be calculated in comparable habitats inside and outside of endemism hotspots and refugia, e.g. across lowland tropical rain forests. Such comparisons facilitate three key implementations for endemism hotspots and refugia: (1) describing functional characteristics, (2) quantifying and comparing the strengths of functional signatures, and (3) evaluating patterns of functional traits, FD and phylogeny to provide a better understanding of eco-evolutionary processes involved in plant community dynamics. These implementations have important applications in biogeography and conservation planning, as outlined below.
Description of the functional characteristics
While scientists agree that endemism hotspots and refugia should be functionally unique from the surrounding landscape (Keppel et al., 2012; Hampe et al., 2013; Harrison and Noss, 2017), no study has demonstrated this comprehensively. Bhagwat and Willis (2008) found that species restricted to high-capacity, southern macrorefugia in Europe during the last glacial maximum have unique functional traits, namely the predominance of long-lived plants with large seeds, which differ considerably from plants believed to have persisted in northern microrefugia (Mosblech et al., 2011; Table 1). These insights highlight the importance of improving our understanding of how functional characteristics shape endemism hotpots and different types of refugia.
Functional characteristics should be linked to the phylogeny of species in the communities, as this can provide additional insights. For example, in the campo rupestre, diversity in dormancy strategies was predominantly found near the base of the phylogenetic tree, suggesting that dormancy traits were strongly selected for in this refugium (Dayrell et al., 2017). Furthermore, ignoring the evolutionary effects on traits can lead to false conclusions (Li and Ives, 2017), as can focusing solely on phylogenetic patterns (Costion et al., 2015).
Comparing the strengths of functional signatures
The strength of functional signatures, i.e. the magnitude of differences in functional traits, can be compared between endemism hotspots or refugia and the surrounding landscape (Fig. 2). As a first step, it is important to determine which aspects (i.e. functional traits, FD, phylogeny) of the functional signature most strongly differentiate the habitats. The functional signatures of different endemism hotspots and refugia can then be compared by focusing on the magnitude of differences in these characteristics.
The strength of the functional signature could be used to indicate the capacity of refugia to buffer environmental change and thereby to facilitate the persistence of biota, which is relevant to the prioritization process in conservation planning (Keppel et al., 2015). Assuming that environmentally stable refugia have more distinctive functional characteristics, the functional signature should be an indicator of the capacity of refugia to facilitate the persistence of biodiversity under environmental change. In this context, the magnitude of differences in FD within and outside refugia, and among different refugia, should be particularly informative, as FD is strongly correlated with functional resilience (Hooper et al., 2005). However, few refugia can provide protection under opposing environmental trends (e.g. cooler and warmer climates; Mokany et al., 2017). Therefore, caution must be exercised when projecting the capacity of refugia into future climates that are novel for the region under consideration.
Using functional signatures to infer and predict eco-evolutionary processes of community assembly
Integrating patterns of FD (i.e. trait convergence and divergence), and potentially FRed, with phylogenetic data can provide a broader view about ecological and evolutionary processes in plant communities of endemism hotspots and refugia (Lavorel and Garnier, 2002; Molina-Venegas et al., 2015; de Bello et al., 2017; Harrison and Noss, 2017). Analyses of functional trait patterns can be cautiously used to infer the prevalent ecological mechanisms shaping community assembly, such as habitat filtering, niche differentiation and facilitation (de Bello et al., 2012; Spasojevic and Suding, 2012; Spasojevic et al., 2014), and to quantify the relative role of resilience (Rosenfeld, 2002; Hooper et al., 2005). Additionally, combining trait data and phylogenetic patterns can inform about evolutionary effects on traits (Blomberg et al., 2003; Kooyman et al., 2011; Molina-Venegas et al., 2015), providing important information about the history and functioning of endemism hotspots and refugia (Dayrell et al., 2017; Harrison and Noss, 2017; Fig. 2).
The proposed eco-evolutionary functional signature approach to the study of endemism hotspots and refugia is also potentially powerful for making predictions about the likely impacts of projected environmental change on plant species, traits and communities. For example, the grasslands of the Brazilian campo rupestre have experienced long-term stability (Silveira et al., 2016) in edaphic conditions (extremely nutrient-poor soils), climate (arid and seasonal precipitation) and disturbance (predictable and frequent fire regime). Plant species and communities in these grasslands (1) are highly stress-tolerant (Negreiros et al., 2014), (2) invest little in seed production with low seed dormancy and (3) are phylogenetically distantly related (Dayrell et al., 2017).
The campo rupestre is projected to experience increasing aridification and altered fire regimes, with some of these changes occurring relatively rapidly. While the trait conservatism associated with long-term stability in the campo rupestre may enhance ecosystem resilience, adaptations to predictable, but stressful, environmental conditions may increase the vulnerability of the flora to collapse under abrupt, swift climate changes (Díaz et al., 2013; Cornelissen and Makoto, 2014; Dayrell et al., 2017). Including additional traits that are informative about above- and below-ground plant strategies related to nutrient and water acquisition, retention and use (e.g. specific leaf area, fine roots, mycorrhizal associations), as well as to resource storage and resprouting ability (e.g. bark thickness, bud bank, rhizomes), could provide valuable information (Pausas et al., 2018). These functional characteristics have been shown to be highly relevant in the Southwest Australian biodiversity hotspot that experiences similar environmental pressures (Enright et al., 2014; Zemunik et al., 2015; Silveira et al., 2016).
CONCLUDING REMARKS
We have provided a conceptual operational framework that facilitates the integration of plant functional traits in studies of endemism hotspots and refugia. This approach allows the systematic integration of ecological characteristics (i.e. functional traits) and evolutionary information (i.e. phylogenetic data) when studying these entities, addressing a major gap in studies of endemism hotspots and refugia (Hampe et al., 2013; Harrison and Noss, 2017). Despite the existing limitations (i.e. lack of trait data, issues in disentangling ecological processes from trait patterns), implementing the proposed eco-evolutionary framework will advance our understanding of endemism hotspots and refugia – especially of the processes shaping biotic patterns. This should assist in predicting the effects of environmental change on these entities, which is likely to be the most important application of our framework, given the pervasive impacts of climate change (Pecl et al., 2017) and the relevance of endemism hotspots and refugia as safe havens for biodiversity (Keppel et al., 2012; Harrison and Noss, 2017).
ACKNOWLEDGEMENTS
We thank Etienne Laliberté (University of Montreal, Canada), Jodi Price (Charles Sturt University, Australia), Andy Gillison (Center for Biodiversity Management, Yangaburra, Australia), Marko J. Spasojevic (University of California Riverside, USA) and Camilla Wellstein (Free University of Bozen, Italy) for insightful comments on early versions of the manuscript. We also acknowledge the helpful comments by Mick Ashcroft (University of Wollongong), one anonymous reviewer and Trude Schwarzacher (handling editor). The authors acknowledge the following funding sources: an ARC Linkage Project (LP0990914) supported by the Australian Research Council, Alexander von Humboldt Fellowship for experienced researchers to GK; Endeavour Europe Award (Australian Government), CIPRS (Curtin University), SIRF, UPAIS, UWA Safety-Net To-Up, and Ad-Hoc scholarship schemes (The University of Western Australia) to GO; Iluka Chair in Vegetation Science and Biogeography at The University of Western Australia, and an ARC linkage project to LM; NASA grant 3-V4B3111 and the Pacific Southwest Regional Center of Excellence for Vector-Borne Diseases funded by the U.S. Centers for Disease Control and Prevention (Cooperative Agreement 1U01CK000516) to MM.
LITERATURE CITED
Author notes
These authors contributed equally to this paper.