-
PDF
- Split View
-
Views
-
Cite
Cite
Ayufu Yilamujiang, Michael Reichelt, Axel Mithöfer, Slow food: insect prey and chitin induce phytohormone accumulation and gene expression in carnivorous Nepenthes plants, Annals of Botany, Volume 118, Issue 2, August 2016, Pages 369–375, https://doi.org/10.1093/aob/mcw110
- Share Icon Share
Abstract
Background and Aims Carnivorous Nepenthes plants use modified leaves forming pitfall traps to capture and digest prey, mainly insects, for additional nutrient supply. These traps, so called pitchers, contain a plant-derived fluid composed of many hydrolytic enzymes and defence-related proteins. In this study, the prey-induced induction of corresponding genes of those proteins and a role for phytohormones in this process was analysed.
Methods Tissue from insect prey-fed, chitin- and phytohormone-challenged pitchers was harvested and analysed for selected gene expressions by a quantitative PCR technique. Phytohormone levels were determined by LC-MS/MS. Nepenthesin proteolytic activities were measured in the digestive fluid using a fluorescence substrate.
Key Results Insect prey in the pitchers induced the accumulation of phytohormones such as jasmonates as well as the transcription of studied genes encoding a chitinase 3 and a protease (nepenthesin I), whereas a defence-related protein (PR-1) gene was not induced. Treatment with chitin as a component of the insects’ exoskeleton triggered the accumulation of jasmonates, the expression of nepenthesin I and chitinase 3 genes similar to jasmonic acid treatment, and induced protease activity in the fluid. All detectable responses were slowly induced.
Conclusions The results suggest that upon insect prey catch a sequence of signals is initiated: (1) insect-derived chitin, (2) jasmonate as endogenous phytohormone signal, (3) the induction of digestive gene expression and (4) protein expression. This resembles a similar hierarchy of events as described from plant pathogen/herbivore interactions, supporting the idea that carnivory evolved from plant defences.
INTRODUCTION
Carnivory in plants is an adaptation for nutrient-poor environments. Different species of carnivorous plants developed various strategies to obtain additional nutrients from caught insect prey, such as sticky flypaper traps in Drosera species or snap traps in Dionaea muscipula (Juniper et al., 1989). Plants of the genus Nepenthes have so-called pitcher traps, representing metamorphosed leaves. Pitchers can be divided into three zones: at the top a peristome that is involved in attracting and trapping the prey; second a slippery waxy zone on the inner side of the pitchers that is involved in trapping and preventing prey escape; and finally, at the bottom the digestive zone which is covered inside with bifunctional glands and contains a digestive fluid. On the one hand, the bifunctional glands secrete hydrolytic enzymes into the fluid and on the other hand they take up the nutrients which are generated by prey digestion in the digestive fluid (Mithöfer, 2011).
There has been a long history of interest in the function and composition of digestive pitcher fluids and some pioneer suggestions about their hydrolytic properties were reported by Charles Darwin (Darwin, 1875). Various hydrolytic proteins have been identified meanwhile, for some of which their corresponding genes were cloned, heterologously expressed and further characterized (Mithöfer, 2011). The most prominent proteins in the pitcher fluid of Nepenthes are two pepsin-related aspartic proteases, nepenthesin I and II, which cleave peptides at the carboxyl side of aspartic acid residues. Nepenthesins were purified, cloned and biochemically characterized from different Nepenthes species (An et al., 2002; Athauda et al., 2004; Takahashi, 2007; Buch et al., 2015). These proteins exhibit stable enzymatic activity up to 60 °C and low pH, which enables the use of nepenthesins for technical applications (Kadek et al., 2014). Besides enzymes with hydrolytic activities, some proteins found in the pitcher fluids of Nepenthes are known to have antimicrobial properties, such as thaumatin-like protein (Rottloff et al., 2009) or the pathogenesis-related protein-1 (PR-1) (Buch et al., 2014). These two examples represent typical pathogenesis-related (PR) proteins with well-known function in plant defence reactions against microbial pathogen attack (van Loon et al., 2006). Strikingly, many of the hydrolytic enzymes in the pitcher fluid are classified as PR proteins as well, for instance chitinases, proteases and glucanases, suggesting that carnivory in plants has evolved from existing defensive reactions.
In recent years, most studies on Nepenthes pitcher fluid have focused on elucidation of the protein composition and they contributed significantly to our knowledge and understanding of prey digestion in these carnivorous plants. However, our knowledge of the molecular regulation of protein secretion in Nepenthes remains limited.
Given that most of the identified pitcher fluid proteins belong to one of the PR protein families (Mithöfer, 2011), it is tempting to speculate that their gene expressions might be initiated by signals derived from caught prey. Furthermore, the endogenous regulation might be mediated by defence-related phytohormones such as jasmonates [jasmonic acid, JA; jasmonic acid–isoleucin conjugate, JA-Ile; cis-(+)-12-oxophytodienoic acid, cis-OPDA] and salicylic acid (SA), similar to the situation in which PR-protein-encoding genes are up-regulated upon pathogen attack. Based on this hypothesis, in this study we investigated the induction of gene expression of selected PR proteins upon insect prey treatment and the role of related phytohormones.
MATERIAL AND METHODS
Plant material and treatment
Nepenthes alata Blanco plants were grown in growth chambers at 20–25 °C, 80–85 % humidity, with a 16-h light/8-h dark photoperiod. To avoid any contamination, 2-week-old and still closed pitchers were used for chitin and phytohormone injections as closed pitcher fluid is known to be free from microbes (Buch et al., 2013). One milligram of colloidal chitin in 500 μL water or only water as a control was injected into the pitcher using sterile pipette tips. For phytohormone-induced gene expression experiments, JA (200 μm final concentrations in the pitcher fluid) or water for controls was injected. For fruit fly (Drosophila melanogaster) treatment pitchers were covered with gauze when they were still closed. Covered pitchers were fed with wild-type D. melanogaster after 2 weeks of pitcher opening according to Buch et al. (2015). In all experiments where phytohormones or gene inductions were analysed in the pitcher tissue, an individual pitcher (biological replicate) was used for one measurement. At least three biological replicates, depending on the availability of utilizable pitchers, were taken for one data point. To analyse JA-Ile directly in the pitcher fluid, 100 μL of pitcher fluid was taken out for each time point (0, 2, 4, 6, 8, 10, 24, 30, 48, and 72 h) after fruit fly treatment. The removed volume was replaced with 25 mm KCl, according to Buch et al. (2013).
Expression analysis by real-time PCR
Tissue from the digestive zone of the pitcher was harvested and frozen in liquid nitrogen and stored in − 80 °C until used. For analysis, frozen plant material was homogenized for 40 s at 1200 r.p.m. in a Genogrinder 2010 (Spex Sample Prep, Stanmore, UK). Total RNA was isolated using an Invitrap Spin Plant RNA Mini kit (STRATEC Molecular, Berlin, Germany) according to the manufacturer’s protocol. First-strand cDNA synthesis was performed using Superscript III reverse transcriptase (Invitrogen, Karlsruhe, Germany) by using Oligo (dT)12–18 and according to the manufacturer`s protocol. For expression analysis by real-time PCR, actin was used as a housekeeping gene by using the following primers: forward primer, 5′-CTCTTAACCCCAAAGCAAACAGG-3′; and reverse primer, 3′-GTGAGAGAACAGCCTGGATG-5′. Nepenthes alata aspartic protease nepenthesin, Nep I (forward primer, 5′-CGGGCAAAAACTTAACCAAA-3′; reverse primer, 3′-ATCCATGATTGCGGAGAAAG-5′); N. alata class 3 chitinase, Chit3 (forward primer, 5′-GCTCCGGCATAGCAGTCTAC-3′; and reverse primer, 3′-CTTGGTTTTGGCATGAGGTT-5′); N. mirabilis pathogenesis-related protein-1, PR-1 (forward primer, 5′-GCCATTGGTATCATCCAACC-3′; and reverse primer, 3′-AGAGCATAAGCCGCAACAGT-5′). Real-time PCR for gene expression analysis was performed in 96-well plates on a Bio-Rad CFX96 Touch Real-Time PCR Detection System (Bio-Rad, Hercules, CA, USA) by using Brilliant II QPCR SYBR green Mix (Agilent, Böblingen, Germany). In total, 60 ng cDNA was used for each sample with a total reaction volume of 25 μL. Obtained mRNA levels of the gene of interest were normalized with respect to the actin mRNA level of each sample, according to Rottloff et al. (2011). Calculations of expression ratios of target genes were related to the mRNA level of target genes in control tissue, which were defined as 1·0; this calculation was done according to Pfaffl (2001). To ensure that gene expression levels were comparable at all different time points, the particular Δct values for the controls of each target gene related to actin gene expression were determined. Only sets of experiments with an s.d. of ≤ 5 % were used for further calculations. All assays were run at least in independent triplicates (biological replications), each biological replicate consisting of three technical replicates.
Nepenthesin protease activity measurement in pitcher fluid
Proteolytic nepenthesin activity in the pitcher fluid was measured by using the specific fluorescence resonance energy transfer-based substrate PFU-093, as described (Kaman et al., 2011; Buch et al., 2015). Briefly, 50 μL of control or induced pitcher fluid, 39 μL water and 1 μL of 80 μm fluorescence substrate PFU-093 were mixed in black 96-well microtitre plates (Greiner Bio-one GmbH, Frickenhausen, Germany). Plates were incubated for 5 h at room temperature. After incubation, 10 μL of 100 mm Tris-HCl buffer, pH 8·5, was added to the mixture before measurement. Fluorescence of cleaved substrate was measured, at 42 °C using a microplate reader (Tecan infinite M200, Männedorf, Switzerland) with excitation/emission wavelength of 485/530 nm, respectively.
Quantification of phytohormones
Phytohormone analyses were carried out according to Vadassery et al. (2012) with some modifications. Thus, harvested plant material (digestive zone of the pitcher) was frozen with liquid nitrogen and stored at − 80 °C until used. For analysis plant material was finely ground in liquid nitrogen and weighed. Ground plant material (250 mg) was extracted with 1·5 mL of methanol containing internal standard: 60 ng of D6-jasmonic acid (HPC Standards GmbH, Cunnersdorf, Germany), 60 ng D4-salicylic acid (Sigma-Aldrich, St Louis, MO, USA) and 12 ng of JA–13C6-isoleucine conjugate which was synthesized as described by Kramell et al. (1988). The homogenate was mixed for 30 min, centrifuged at 14^000 r.p.m. for 10 min at 4 °C, subsequently re-extracted with 500 μL methanol and both supernatants were pooled. The combined extract was dried, re-dissolved in 500 μL methanol and used for chromatography. For analysis of pitcher fluid, the fluid was spiked with 2 ng mL–1 of fluid for JA–13C6-isoleucine conjugate and used for chromatography. Separation was performed on an Agilent 1200 HPLC system (Agilent Technologies, Santa Clara, CA, USA) equipped with a Zorbax Eclipse XDB-C18 column (50 × 4·6 mm, 1·8 µm; Agilent). Formic acid (0·05 %) in water and acetonitrile were employed as mobile phases A and B, respectively. The elution profile was: 0–0·5 min, 5 % B; 0·5–9·5 min, 5–42 % B; 9·5–9·51 min, 42–100 % B; 9·51–12 min, 100 % B; and 12·1–15 min, 5 % B keeping a flow rate of 1·1 mL min–1. Column temperature was maintained at 25 °C. An API 5000 tandem mass spectrometer (Applied Biosystems, Foster City, CA, USA) equipped with a Turbospray ion source was operated in negative ionization mode. Ion spray voltage was maintained at − 4500 eV, turbo gas temperature was set at 700 °C, nebulizing gas was set at 60 p.s.i., curtain gas at 25 p.s.i., heating gas at 60 p.s.i. and collision gas at 7 p.s.i. To monitor analyte parent ion → product ion, multiple reaction monitoring (MRM) was used: m/z 209·1 →59·0 (collision energy (CE) − 24 V; declustering potential (DP) − 35 V) for JA; m/z 215·1 → 59·0 (CE − 24 V; DP − 35 V) for D6-JA; m/z 136·9 → 93·0 (CE − 22 V; DP − 35 V) for SA; m/z 140·9 → 97·0 (CE − 22 V; DP − 35 V) for D4-SA; m/z 290·9 → 165·1 (CE − 24 V; DP − 45 V) for cis-OPDA, m/z 322·2 → 130·1 (CE − 30 V; DP − 50 V) for JA-Ile conjugate; m/z 328·2 → 136·1 (CE − 30 V; DP − 50 V) for JA–13C6-Ile conjugate. Both Q1 and Q3 quadrupoles were maintained at unit resolution. Analyst 1.5 software (Applied Biosystems) was used for data acquisition and processing. Phytohormones were quantified relative to the signal of their corresponding internal standard. For quantification of cis-OPDA, D6-JA was used as the internal standard applying an experimentally determined response factor of 0·5.
RESULTS AND DISCUSSION
Fruit-fly-induced PR gene expressions and phytohormone accumulation
Carnivorous plants of the genus Nepenthes digest insect prey in the pitcher trap fluid to supplement their nutrient demand. The pitcher fluid is present in the bottom part of the trap and its composition is supplied by secretion processes via specific glands. To study insect prey-induced gene expression in the pitcher tissue, three corresponding genes of well-characterized proteins from the pitcher fluid were chosen, namely nepenthesin I (Nep I; Buch et al., 2015), class III chitinase (Chit3; Hatano and Hamada, 2012) and pathogenesis-related protein 1 (PR-1; Buch et al., 2014). Gene expression of the aspartic protease Nep I was slightly but significantly upregulated after 12 and 24 h and strongly after 48 h of fruit fly treatment compared with the control. Chit3 was also slightly upregulated after 24 h and strongly after 48 h. In contrast, PR-1 gene expression did not change (Fig. 1). Because fruit flies represent insect prey, the result obtained can be explained by the fact that both upregulated genes represent enzymes involved in prey digestion. PR-1, by contrast, has no enzymatic but antimicrobial activity (Buch et al., 2014), which might not be necessary to establish at this time point. Furthermore, the result found for Nep I corresponds well to a result that was recently published (Buch et al., 2015), in which the authors showed that after 48 h the enzymatic nepenthesin I activity in the pitcher fluid was strongly increased when fed with fruit flies. In the same study, they showed that nepenthesin I activity was induced by JA treatment alone, but only after several days.
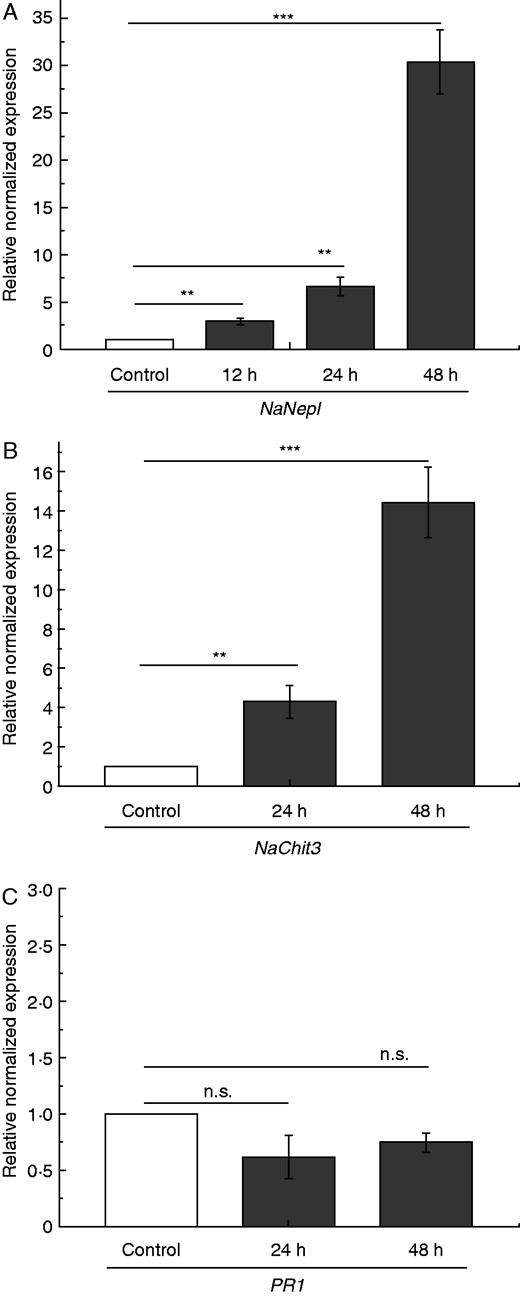
Fruit fly-induced PR gene expression in N. alata pitchers. Pitchers were fed with 60 mg fruit flies and mean expression (± s.e.; n = 3–5) of selected PR genes was analysed. NepI (A) expression was analysed after 12, 24 and 48 h; Chit3 (B) and PR-1 (C) were analysed after 24 and 48 h both in untreated (white) and treated (grey) pitchers. Statistically significant differences between control and treated pitchers were analysed by Student’s t-test for each gene separately. Gene expression for control was set as 1·0; n.s.: not significant, **P < 0·01, ***P < 0·001.
Recently it has been suggested for different carnivorous plants that phytohormones are involved in the digestion process, for example in Drosera capensis (Nakamura et al., 2013) or Venus flytrap (Dionaea muscipula). In the latter case the application of JA induced the abundance of a cysteine protease, dionain, and increased proteolytic activity in the digestive fluid (Libiakova et al., 2014). Escalante-Perez et al. (2011) described the involvement of cis-OPDA in the induction of digestive fluid secretion in D. muscipula. Those results also strongly suggest a role for phytohormones in the fruit fly-induced process of gene inductions in Nepenthes. To prove this hypothesis, the level of the biologically most active jasmonate, JA-Ile, was first determined in the pitcher fluid of N. alata at various time points after D. melanogaster treatment (Fig. 2). Such an experiment was necessary to gain insight into the kinetics of jasmonate accumulation induced by caught prey. As shown in Fig. 2, when insect prey was added, JA-Ile concentration increased continuously up to 24 h and stayed at that level, indicating a relative slow phytohormone response. One advantage of this experiment was that the samples could be harvested continuously from the pitchers, showing the dynamics of the system; a disadvantage was that phytohormones are generated and active in the plant tissue. However, using one pitcher for one measurement for several biological replicates and numerous time points is simply not feasible as the number of developmentally comparable pitchers is limited. Therefore, the results obtained from the pitcher fluid experiment provided valuable data and the basis for subsequent experiments for the analysis of different phytohormones in the tissue at defined time points. After fruit fly treatment, jasmonates, i.e. JA and JA-Ile as well as their biosynthetic precursor, cis-OPDA, significantly accumulated over time in pitcher tissue (Fig. 3). Also, the SA level was found to be significantly higher after 48 h of fruit fly treatment [0 h: 2·3 ± 0·5 ng SA (g f. wt)–1; 48 h: 8·5 ± 1·9 ng SA (g f. wt)–1; P < 0·005, Student’s t-test]. This indicates that prey catch and the subsequently digestion in Nepenthes is very likely a phytohormone-mediated process, depending at least on jasmonates.
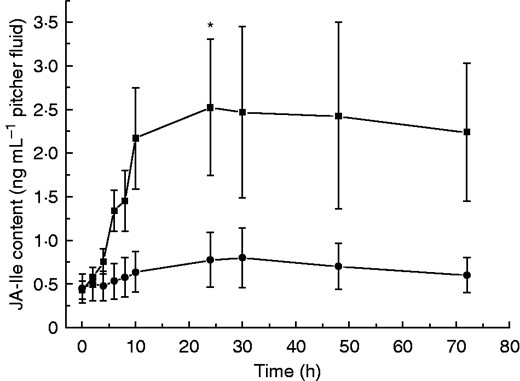
JA-Ile accumulation in fruit fly-fed pitcher fluids of N. alata. Pitchers were fed with 60 mg fruit flies and the level of JA-Ile was analysed after addition of fruit flies at different time points in pitcher fluids. Mean JA-Ile content (± s.e.) was analysed in both control (•) (n = 3) and fruit fly-treated (▪) (n = 7) pitchers. Statistically significant differences between control and treated pitchers were analysed for each time point by Student’s t-test separately; *P < 0·05.
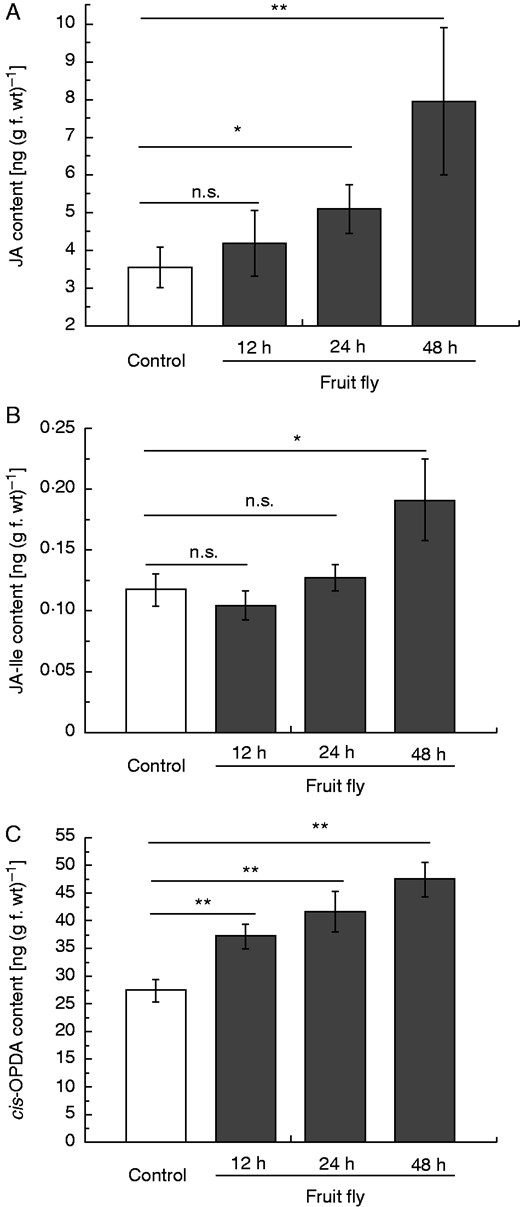
Phytohormone accumulation in fruit fly-fed pitchers of N. alata. Pitchers were fed with 60 mg fruit flies and endogenous phytohormone levels of JA (A), JA-Ile (B) and cis-OPDA (C) were analysed after 12, 24 and 48 h in pitcher tissue. Mean phytohormone content (± s.e.; n = 3–5) was analysed in both untreated control (white) and treated (grey) pitchers. Statistically significant differences between control and treated pitchers were analysed by Student’s t-test for each hormone separately; n.s.: not significant, *P < 0·05, **P < 0·01.
Chitin-induced PR gene expression and phytohormone accumulation
To further investigate whether a certain signal derived from insect prey might be sufficient to induce gene expression and phytohormone accumulation in Nepenthes, chitin as a major component of the insects’ exoskeleton was chosen to mimic caught prey. It has already been reported that in N. khasiana pitchers chitin can induce the accumulation of naphthoquinones (Eilenberg et al., 2010; Raj et al., 2011) as well as gene expression of chitinases, e.g. type NkChit1b (Eilenberg et al., 2006; Hatano and Hamada, 2012). Interestingly, compared with the non-treated control, chitin-induced Nep I gene expression was determined after 24 h; for Chit3 and PR-1 only a trend in the same direction was detected (Fig. 4). After 48 h the situation was different. Nep I gene expression was downregulated to control level but Chit3 transcripts were found to be upregulated; again, PR-1 showed no obvious differences compared with the non-treated control pitchers (Fig. 4). For PR-1 and Chit3 the situation after 48 h resembles the situation found after fruit fly treatment (Fig. 1), suggesting that chitin very likely represents at least one of probably more insect prey-derived signalling compounds that is involved in the induction of downstream events in Nepenthes necessary to initiate the digestion process. However, for Nep I the situation is different. Here, after 48 h challenge with chitin, gene expression is already down again whereas upon fruit fly treatment a high level of transcripts was found. This situation can be explained by the fact that after chitin application this particular signal molecule is present in relatively high concentrations, can act directly and must not be released from the prey. Therefore, it might act faster. Moreover, the much higher induction of Nep I after 48 h upon challenge with D. melanogaster (Fig. 1A) could be due to additional signals that are absent upon sole chitin treatment but might be released slowly from the whole insect prey. Interestingly, when the pitchers were treated with JA (200 μm), a weak Nep I gene induction (1·5-fold; P < 0·05, Student’s t-test) was determined only after 24 h, whereas the Chit3 gene was found to be induced (1·6-fold; P < 0·05, Student’s t-test) only after 48 h, comparable to the induction pattern upon chitin treatment. Together, these results suggest different gene regulation mechanisms among the three selected genes.
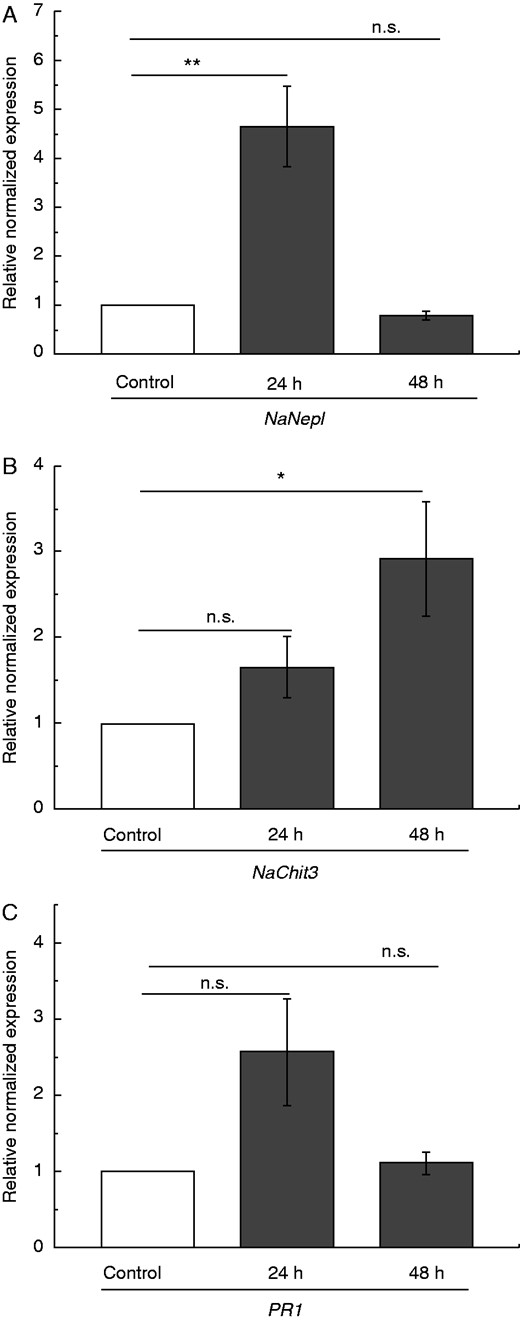
Chitin-induced PR gene expression in pitcher tissue of N. alata. Pitchers were treated with chitin and mean expression (±s.e.; n = 3) of selected PR genes NepI (A), Chit3 (B) and PR-1 (C) was analysed after 24 and 48 h in both untreated (white) and treated (grey) pitchers. Statistically significant differences between control and treated pitchers were analysed by Student’s t-test for each gene separately. Gene expression for control was set as 1·0; n.s.: not significant, *P < 0·05, **P < 0·01.
Based on the former results it was tempting to speculate that chitin can also induce phytohormone accumulation. This was investigated again in comparison with non-treated control pitchers. After 24 h, JA and cis-OPDA only showed a trend to higher concentrations but after 48 h the level of both compounds was significantly enhanced. JA-Ile showed significantly higher concentrations after 24 h and to the same extent after 48 h (Fig. 5B). Strikingly, the basic concentrations of cis-OPDA and JA-Ile, in the latter case less pronounced, were about 1·5- to 2-fold higher in the experiments with chitin treatment (Fig. 5) compared with the fruit fly experiments (Fig. 3). This is probably due to slightly different developmental stages of the pitchers. Whereas the pitchers for fruit fly feeding need to be open, for chitin treatment unopen pitchers were used to avoid any contamination. However, the corresponding controls were always performed to see the difference between non-treated and treated pitchers. SA concentrations did not show any changes after 24 h [0 h: 3·8 ± 0·6 ng SA (g f. wt)–1; 24 h: 3·8 ± 0·7 ng SA (g f. wt)–1; not significant, Student’s t-test] but were found nearly doubled after 2 d of chitin treatment (48 h: 7·1 ± 0·8 ng SA (g f. wt)–1; P < 0·01, Student’s t-test).
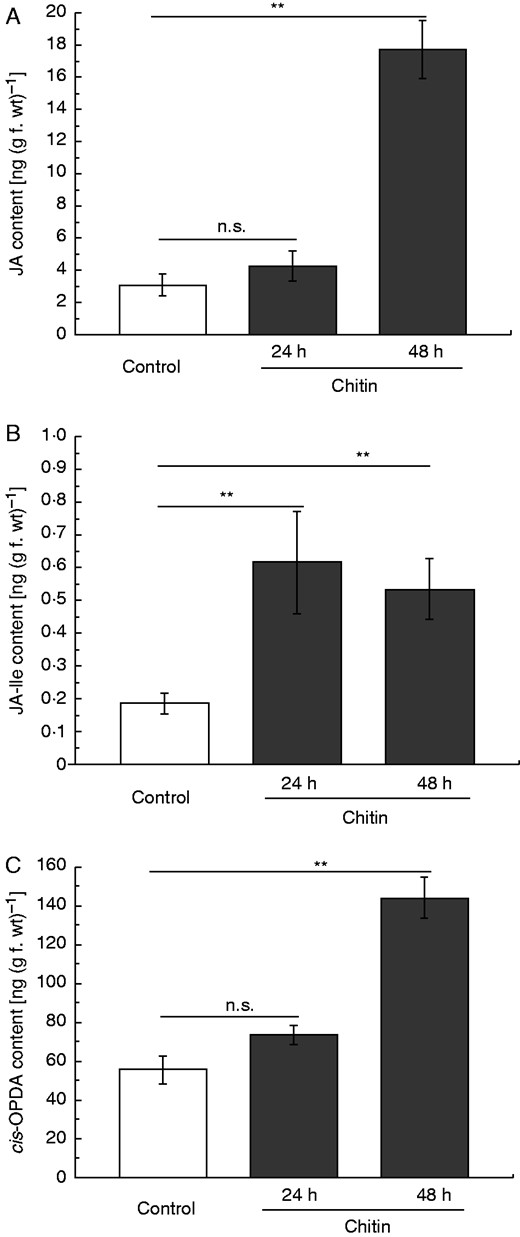
Jasmonate induction in chitin-treated pitcher tissue of N. alata. Pitchers were treated with chitin and endogenous phytohormone levels of JA (A), JA-Ile (B) and cis-OPDA (C) were analysed after 24 and 48 h in pitcher tissue. Mean phytohormone content (±s.e.; n = 3–5) was analysed in both control (white) and treated (grey) pitchers. Statistically significant differences between control and treated pitchers were analysed by Student’s t-test separately; n.s.: not significant, **P < 0·01.
Chitin-induced nepenthesin activity
Gene expression analyses provided evidence that the encoded proteins and corresponding enzymatic activities are probably upregulated as well; in fact, however, this had to be proven. Thus, the proteolytic activity of Nep 1 was exemplarily measured in the digestive fluid upon chitin treatment of the pitchers. Indeed, after 24 and 48 h, significantly higher enzymatic activities were detected compared with the non-treated control pitchers (Fig. 6). Strikingly, again establishment of the enzymatic activity was faster upon chitin than fruit fly treatment (Buch et al., 2015), resembling and supporting the results obtained for gene expression.
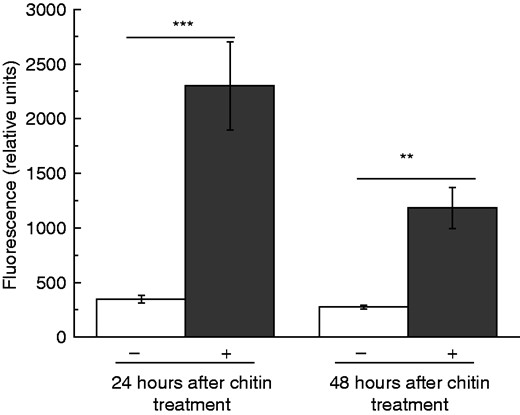
Chitin-induced nepenthesin activity in the pitcher fluid of N. alata. Pitchers were treated with chitin and nepenthesin protease activity in the pitcher fluid was measured by using a fluorescence substrate (PFU-093) at 24 and 48 h after treatment. Mean relative fluorescence (±s.e.; n = 3–4) was analysed in both control (white) and treated (grey) pitchers. Statistically significant differences between control and treated pitchers were analysed by Student’s t-test; **P < 0·01, ***P < 0·001.
CONCLUSIONS
The results presented here support the hypothesis that plant carnivory evolved from plant–herbivore interactions, in particular employing elements of plant defence against herbivorous insects. This includes jasmonates as endogenous signalling molecules, such as JA and JA-Ile, as well as preceding recognition of a prey-related signal. In the case of caught insects in Nepenthes, chitin represents such a signal. The prey-initiated signalling cascade eventually leads to the induction of genes encoding enzymes involved in prey digestion but also classified as PR proteins, participating in plant defence. Thus, another tessera is provided suggesting that the origin of carnivory in plants developed from plant defences. Strikingly, insect-induced phytohormone accumulations, gene expression and enzymatic activities are detectable only with delay, if compared with insect attack-induced defence responses. This suggests that the onset of digestion in carnivorous Nepenthes plants is a comparatively slow process, perhaps because the prey is already caught and there is no need for the plant to hurry.
ACKNOWLEDGEMENTS
We thank Birgit Arnold and the whole greenhouse team at the MPI for Chemical Ecology for growing Nepenthes plants; Floris J. Bikker, Amsterdam, for providing PFU-093 substrate; Silke Trautheim for providing fruit flies; Sandra Scholz for continuous help; and Wilhelm Boland and the Max Planck Society for support.
LITERATURE CITED